© Borgis - Postępy Nauk Medycznych 12/2013, s. 847-855
*Iwona Grabska-Liberek, Katarzyna Chwiejczak, Barbara Terelak-Borys, Jacek Kosmala
Jaskra a choroba Alzheimera – wspólne patomechanizmy i metody terapii
Glaucoma and Alzheimer’s Disease – Common Pathomechanisms and Therapeutic Measures
Clinic of Ophthalmology, Medical Center of Postgraduate Education,
Prof. W. Orłowski Independent Public Clinical Hospital, Warszawa
Head of Clinic: Iwona Grabska-Liberek, MD, PhD, assoc. prof.
Streszczenie
Przez całe życie osobnicze dochodzi równolegle do utraty komórek organizmu i ich regeneracji. Wraz z wiekiem zmniejszają się zdolności regeneracyjne, a przewagę zyskują procesy katabolizmu. Starzenie się jest związane także z utratą komórek nerwowych, postępującą systematycznie i nieprzerwanie aż do śmierci. Jest to zjawisko normalne i nieuniknione, jednak gdy zostanie przekroczony fizjologiczny próg śmierci neuronów, możemy mówić o patologii – chorobie neurodegeneracyjnej. Do tej grupy zalicza się kilka jednostek chorobowych z zakresu neurologii, jak choroba Alzheimera i choroba Parkinsona, a także jaskrę, będącą domeną okulistyki. Na pozór jaskra, wciąż kojarzona powszechnie z podwyższonym ciśnieniem śródgałkowym, pozostaje w dość luźnym związku z pozostałymi wymienionymi jednostkami chorobowymi, jednak okazuje się, że wspólnych cech jest bardzo wiele. Wykazano, że jaskra istotnie częściej występuje u osób dotkniętych chorobą Alzheimera, zaś do zaniku komórek nerwowych w nerwie wzrokowym prowadzą mechanizmy podobne do tych, które obserwuje się w chorobach neurodegeneracyjnych mózgu. W niniejszym artykule podjęto próbę zbiorczego przedstawienia i usystematyzowania dostępnej wiedzy na temat podłoża współistnienia jaskry i choroby Alzheimera. Przedstawiono wyniki badań oraz teorie i hipotezy z zakresu biologii molekularnej, cytofizjologii, patofizjologii, immunologii i farmakologii. Niewątpliwie, zrozumienie patomechanizmów neurodegeneracji stanowi klucz do opracowania nowych sposobów leczenia zarówno jaskry, jak i choroby Alzheimera.
Summary
Throughout the life of an organism, its cells are being lost and regenerated at the same time. With age, the ability to regenerate diminishes, and the catabolic processes are in the ascendant. Normal aging is also associated with the constant loss of the neurons, continuing till death. This phenomenon is natural and inevitable; however, as soon as the physiological threshold is exceeded, the pathology known as a neurodegenerative disease begins. The group embraces not only a number of neurological disorders, like Alzheimer’s disease or Parkinson’s disease, but also glaucoma, an ophthalmic pathology. Contrary to the common knowledge, binding glaucoma to the increased intraocular pressure, the disease has numerous associations with the neurological diseases mentioned above. It has been shown, that glaucoma is significantly more common in the patients with Alzheimer’s disease, and, that the mechanisms leading to the cell death in the optic nerve are very similar to the ones observed in neurodegeneration of the brain. The article analyzes current literature in attempt to review and systematize the knowledge regarding the coexistence of glaucoma and Alzheimer’s disease. The results of the researches, the theories and hypotheses from the fields of the molecular biology, cytophysiology, pathophysiology, immunology and pharmacology were presented. Certainly, the knowledge on the pathomechanisms of neurodegeneration is crucial for finding the new therapeutic methods for glaucoma, as well as Alzheimer’s disease.
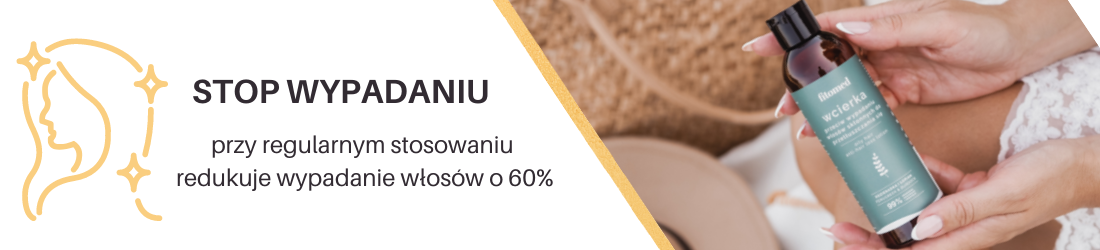
INTRODUCTION
Alzheimer’s disease (AD) is a chronic, multifactorial neurodegenerative disease which irreversibly damages the nerve cells of the cerebral cortex, especially in the region of association neocortex and the hippocampus. This process is a result of pathological mechanisms rather than accelerated physiological ageing. In a normally ageing brain, the metabolic activity is decreased in the area of subiculum and in the dentate nucleus, while the AD is characterized by a lowered activity of the entorhinal cortex. Histopathologically, the AD, unlike physiological ageing, causes a loss of entorhinal and hippocampus field CA1 neurons as well as a decrease in the central temporal lobe volume (1). Other histopathological features of the AD are: extracellular senile plaques composed of β-amyloid and fibrillary degeneration (a fibre network formed by Tau protein neurofibrils) (2, 3). Although these lesions allow a certain diagnosis, they are accessible only through post-mortem examination. Clinical diagnosis of the AD is difficult and often lacks precision as the delay between first symptoms and the diagnosis is usually 2-4 years. Diagnosis and differentiation is carried out on the basis of symptoms, psychological tests results (e.g. Mini-Mental State Examination) and by means of exclusion of organic disorders (4, 5). While mental fitness among healthy population is on constant level with only gradual deterioration of the recent memory and slowing-down of information processing, persons with AD suffer from profound impairment of cognitive functions and different forms of dementia. Cognitive disorders are characterized by memory and speech issues as well as problems with other basic cognitive functions, which are noticeable by others and easily detected during tests but do not impair normal functioning. Whereas dementia is characterized by progressive, generalized decline in cognitive functions in many areas, including memory, and one additional – learning, orientation, speech, understanding and ability of evaluation, which is profound enough to hinder normal functioning. Over time, AD persons lose the ability to perform the most basic activities like verbal communication, moving, food intake or sphincter control (5, 6). In the USA, AD is the 7th most common cause of death and 5th most common among people of 65 and more years old. Only in the USA 5.3 million people suffer from AD (7). It is estimated that in the age group of 65-74 years old, 5-10% of the population is affected by dementia while in people over 85 years old it is 25-50%. Alzheimer’s disease is the most commonly occurring neurodegenerative disease and, depending on the criteria applied, consists of 50-80% of dementia cases. The hereditary form of this disease, connected with mutations of the amyloid precursor protein, presenilin 1 and 2 as well as of apolipoprotein ?ε4, occurs in only 5% of patients. In other cases it is of late-onset sporadic form.
Glaucoma is described as a disease of the optic nerve with distinctive lesions in the optic disc and typical changes in the field of vision with or without heightened intraocular pressure (IOP) (8). Therefore, intraocular pressure is of no diagnostic value for glaucoma. This is because 20-30% of glaucoma patients have IOP within normal limits i.e. typically 10-20 mmHg (9). It has also been established that intraocular pressure-lowering treatment does not guarantee a stabilization of the disease, which progress remains on 9-10% level per year, while in patients treated pharmacologically or surgically the disease progresses 20-25% (10, 11).
Glaucoma is the second most common cause of blindness in the world (12). According to the estimations by WHO, there are 66 million people living with glaucoma and approx. 12.5 million lost their vision due to this disease. These numbers are likely to increase along with demographic changes (8). With such devastating statistics, the information that despite intensive research, lowering the IOP is the only available therapeutic tactic is quite pessimistic (8, 13). Although heightened IOP is an important glaucoma progression risk factor, it is not the only one, others being age, binocular disease, pseudoexfoliation syndrome (PEX) and lower arterial systolic pressure (14).
The main element in the pathophysiology of glaucoma is the loss of retinal ganglion cells (RGCs) and their axons over many years. It leads to changes in the morphology of the optic disc and consequently to visual field defects, detectable when 40% of RGCs are already irreversibly lost (12, 15). It may happen that before a patient notices visual field defects, 90% of RGCs are lost (8). These cells are extremely susceptible to damage due to their intensive metabolism. To visualise this, let’s imagine a cell body of a RGC enlarged to the size of an apple – the neuron would be 800 meters long! Energy expenditures are increased by the lack of myelin sheath from the retina to the cribrum and meeting those expenditures is rendered difficult by the structure of the blood vessels which are thin and of relatively rare texture. This makes the neurons susceptible to damaging factors like metabolic stress (hypoxia), free radicals, mechanical pressure or photooxidative damage (16).
RGCs die through apoptosis (although in a more advanced disease necrosis can also take place) with main regulators being protease enzymes – caspases. They are activated endogenously (connected with mitochondrial mediators) and exogenously (activated by extracellular ligands like TNF-α). Changes in the retinal extracellular matrix as well as activation of metalloproteinases may occur as a reaction to the heightened IOP, which disturbs the cell-cell and cell-extracellular matrix reactions and triggers a cascade of reactions leading to apoptosis. Heightened IOP may also disturb the transportation of growth factors, i.a. BDNF (brain-derived neurotrophic factor), and stimulate the synthesis of others e.g. TGFβ2. Circulatory insufficiency may also play an important role through direct damaging, as well as stimulation of apoptosis. Glutamate released by retinal cells as a reaction to hypoxia can also have a significant impact. It acts through several ionotropic receptors (NMDA, KA, AMPA) and metabotropic receptors (mGluR) causing an influx of Ca2+ to the cell, activating second transmitters and, as a result, death. A toxic influence of nitric oxide (NO) which is a product of synthetase 2 (NOS-2) has also been demonstrated (12). Other factors causing apoptosis are: dysfunction of mitochondria and oxidative stress connected with it and protein synthesis disorders – accumulation of β-amyloid (17). Some of the research show the role of immune system in pathophysiology of glaucoma (18). This long and still incomplete list of possible mechanisms in which the death of RGCs takes place proves how significant and important the problem of optic nerve degeneration is in glaucoma. It has also been proven that lowering IOP by 20% does not improve the functioning of RGCs (19).
COEXISTENCE OF GLAUCOMA AND ALZHEIMER’S DISEASE
Up to this point, many studies have been carried out to irrefutably ascertain the neurodegenerative nature of glaucoma, but the first one in this direction was conducted by Hinton, Sadun et al. in 1986. They compared post-mortem the optic nerves of AD persons with nerves of healthy people of similar age and discovered that 8/10 AD persons had an extensive axonal degeneration of optic nerves, much different from changes related to normal aging (20). Later, Sadun and Bassi published the results of their research in which they determined the degeneration of RGCs and their axons in post-mortem histopathological examination of AD persons’ eyes. It mostly concerned largest M cells and was not observed in the control group.
Based on these results, Bayer, Ferrari and Erb conducted an examination on 112 AD patients from Upper Bavaria in Germany. Glaucoma was diagnosed in 25.9% persons while no ocular hypertension was observed. In the control group, glaucoma was identified in 5.2% of patients only and OHT in 7.8%. Thus, a conclusion was drawn that a risk of glaucoma is greater for AD persons and also that their optic nerves are more susceptible to heightened IOP (21). Similar observations were performed by Tamura et al. in Japan, where they studied the incidence of primary open-angle glaucoma in AD persons. Glaucoma was diagnosed in 23.8% of 172 persons examined, occurring much frequently than in the control group (9.9%) and in average Japan population (7.6%). They also analysed the frequency of occurrence of the APOEε4 allele, apolipoprotein ε4 gene, however all they could prove was that APOEε4 occurs more often in AD persons which was of no relevance to the incidence of glaucoma (22). These studies show that more frequent incidence of glaucoma in AD persons is not racially dependent. At the same time, the thesis of increased incidence of AD in primary open-angle glaucoma persons was not confirmed (23).
PSEUDOEXFOLIATION SYNDROME (PEX)
Linnér et al. tried to prove the connection between PEX-related glaucoma and dementia, based on premises that PEX is a systemic disease which also manifests in AD as protein deposits in brain. Although the incidence of PEX in dementia persons was significant – 28.8%, it was not linked to the incidence of AD. The incidence of PEX was even greater in patients with vascular dementia. This may be explained by difficult differentiation between vascular lesions and AD, but the study makes drawing definite conclusions difficult (24).
β-AMYLOID
These are not the only attempts to link glaucoma and AD as finding such connection may open new diagnostic and therapeutic possibilities. I will focus on β-amyloid (Aβ). Although its role in AD is still discussed, it is known that senile plaques play an important role in pathophysiology of AD. They are composed of Aβ which is formed by polymerizing peptides stemming from amyloid precursor protein (APP) The ones which most easily aggregate and are the most toxic are those containing 42 first amino acids – the isoform Aβ42. They damage the synaptic transport by blocking synaptic receptors, impairing ion transport and having a toxic effect on nerve cells, what damages mitochondria and activates apoptosis through caspases (25, 26). In experiments performed on animal models of glaucoma, increased levels of Aβ and caspases were observed: 3. and 8. in RGCs layer and in the optic nerve of eyes with high IOP (27, 28). In other studies, an Aβ fragment (25-35) was neurotoxic both to nerve cells of cerebral cortex as well as to RGCs (29, 30).
These findings became the basis for the development of new methods for treatment of glaucoma which aim at blocking the synthesis and aggregation of Aβ. Although the tests of these potential drugs are on experimental level, the results are promising. In an experiment on rats, 3 substances were used: 1) β-secretase inhibitors decreasing the formation of Aβ, 2) anti-Aβ antibody, 3) Congo red which inhibits aggregation and neurotoxicity of Aβ. All three methods proved very successful (anti-Aβ antibody in particular), slowing down the apoptosis of RGCs and decreasing its intensity. However, truly spectacular results were achieved by combining of these 3 preparations – apoptosis was reduced by 84% (31). Considering that Aβ is inseparably linked with AD, the results described may be of use in the treatment of this disease as well.
GLAUCOMA AND ALZHEIMER’S DISEASE AS TAUOPATHIES
A different protein-tau-plays a documented role in the pathology of AD. Physiologically, tau protein stabilizes the cytoskeleton of neurons, although in AD it undergoes hyperphosphorylation, creating paired helical filaments and causing neurofibrillary degeneration. Hyperphosphorylated tau disintegrates microtubules and aggregates normal tau, MAP proteins 1 and 2 and ubiquitin, creating neurofibrillary tangles. These insoluble structures damage the functions of cytoplasm and impair axonal transport which can lead to cell’s death (32). Pathologic form of tau protein was found during an examination of intra-operative samples collected from glaucoma patients, whereas it was not found in the control group. Particularly high level was registered in retinal horizontal cell (33).
Studies of tau protein are currently being conducted. Therapies differ: some inhibit kinases connected with tau protein, other modify the formation of microtubules. Recently, methylene blue, which blocks the aggregation of tau, has passed the 2nd phase of trials. Eighty-one per cent slow-down of AD symptoms progression in treated persons, in comparison with the control group, was observed (34). Maybe this type of neuroprotection of the optic nerve, although methylene blue dyes the sclera in blue, will find use as well?
HEAT SHOCK PROTEINS
One more matter connected with protein synthesis is worth raising. Heat shock proteins (HSP) prevent the aggregation of denatured proteins and serve as chaperones, facilitating protein folding, changing and restoring normal protein conformation and transmembrane transport. The role of HSP-70 and its ATPase was studied, with a conclusion that the induction of HSP-70 connected with inactivation of ATPase can be effective in AD treatment (35). An increased level of HSP-60 and HSP-27 in glaucoma eyes was observed along with an increased level of anti-HSP antibodies in blood. Whereas geranylgeranylacetone, through an increase of HSP-72 level in RGCs, protected cells against glaucomatous damage. The drug, although not clinically trialled yet, seems to be promising because of its low toxicity and possibility of receiving it per os (16).
GLUTAMATE EXCITOTOXICITY
Powyżej zamieściliśmy fragment artykułu, do którego możesz uzyskać pełny dostęp.
Mam kod dostępu
- Aby uzyskać płatny dostęp do pełnej treści powyższego artykułu albo wszystkich artykułów (w zależności od wybranej opcji), należy wprowadzić kod.
- Wprowadzając kod, akceptują Państwo treść Regulaminu oraz potwierdzają zapoznanie się z nim.
- Aby kupić kod proszę skorzystać z jednej z poniższych opcji.
Opcja #1
29 zł
Wybieram
- dostęp do tego artykułu
- dostęp na 7 dni
uzyskany kod musi być wprowadzony na stronie artykułu, do którego został wykupiony
Opcja #2
69 zł
Wybieram
- dostęp do tego i pozostałych ponad 7000 artykułów
- dostęp na 30 dni
- najpopularniejsza opcja
Opcja #3
129 zł
Wybieram
- dostęp do tego i pozostałych ponad 7000 artykułów
- dostęp na 90 dni
- oszczędzasz 78 zł
Piśmiennictwo
1. Bishop NA, Lu T, Yankner BA: Neural mechanisms of ageing and cognitive decline. Nature 2010; 464: 529-535.
2. Mancuso M, Orsucci D, LoGerfo A et al.: Clinical features and pathogenesis of Alzheimer’s disease: involvement of mitochondria and mitochondrial DNA. Adv Exp Med Biol 2010; 685: 34-44.
3. Maccioni RB, Muñoz JP, Barbeito L: The molecular bases of Alzheimer’s disease and other neurodegenerative disorders. Arch Med Res 2001; 32: 367-381.
4. Schmitt FA, Wichems CH: A Systematic Review of Assessment and Treatment of Moderate to Severe Alzheimer’s Disease. Prim Care Companion J Clin Psychiatry 2006; 8: 158-159.
5. Kłoszewska I: Choroba Alzheimera. Przew Lek 2001; 4: 78-82.
6. Daviglus ML, Bell CC, Berrettini W et al.: National Institutes of Health State-of-the-Science Conference Statement: Preventing Alzheimer Disease and Cognitive Decline. Ann Intern Med 2010; 153: 176-181.
7. Alzheimer’s Association: 2010 Alzheimer’s disease facts and figures. Alzheimers Dement 2010; 6: 158-194.
8. Guideline Development Group: Glaucoma: diagnosis and management of chronic open angle glaucoma and ocular hypertension. London: the National Collaborating Centre for Acute Care, 2009 guideline.
9. Sommer A, Tielsch JM, Katz J et al.: Relationship between intraocular pressure and primary open angle glaucoma among white and black Americans. The Baltimore Eye Survey. Arch Ophthalmol 1991; 109: 1090-1095.
10. Musch DC, Gillespie BW, Lichter PR et al.: Visual field progression in the Collaborative Initial Glaucoma Treatment Study the impact of treatment and other baseline factors. Ophthalmology 2009; 116: 200-207.
11. Seong GJ, Rho SH, Kim CS et al.: Potential benefit of intraocular pressure reduction in normal-tension glaucoma in South Korea. J Ocul Pharmacol Ther 2009; 25: 91-96.
12. Agarwal R, Gupta SK, Agarwal P et al.: Current concepts in the pathophysiology of glaucoma. Indian J Ophthalmol 2009; 57: 257-266.
13. The Advanced Glaucoma Intervention Study (AGIS): The relationship between control of intraocular pressure and visual field deterioration. Am J Ophthalmol 2000; 130: 429-440.
14. Leske MC, Heijl A, Hyman L et al.: Predictors of long-term progression in the early manifest glaucoma trial. Ophthalmology 2007; 114: 1965-1972.
15. Rohit V, Peeples P, Walt JG, Bramley TJ: Disease Progression and the Need for Neuroprotection in Glaucoma Management. Am J Manag Care 2008; 14: 15-19.
16. Schmidt KG, Bergert H, Funk RH: Neurodegenerative diseases of the retina and potential for protection and recovery. Curr Neuropharmacol 2008; 6: 164-178.
17. Cheung W, Guo L, Cordeiro MF: Neuroprotection in glaucoma: drug-based approaches. Optom Vis Sci 2008, 85: 406-416.
18. Gülgün T, Wax MB: Glaucoma. Chem Immunol Allergy 2007; 92: 221-227.
19. Hinton DR, Sadun AA, Blanks JC, Miller CA: Optic-Nerve Degeneration in Alzheimer’s Disease. N Engl J Med 1986; 315: 485-487.
20. Sadun AA, Bassi CJ: Optic nerve damage in Alzheimer’s disease. Ophthalmology 1990; 97: 9-17.
21. Bayer AU, Ferrari F, Erb C: High occurrence rate of glaucoma among patients with Alzheimer’s disease. Eur Neurol 2002; 47: 165-168.
22. Tamura H, Kawakami H, Kanamoto T et al.: High frequency of open-angle glaucoma in Japanese patients with Alzheimer’s disease. J Neurol Sci 2006; 246: 79-83.
23. Kessing LV, Lopez AG, Andersen PK, Kessing SV: No increased risk of developing Alzheimer disease in patients with glaucoma. J Glaucoma 2007; 16: 47-51.
24. Linnér E, Popovic V, Gottfries CG et al.: The exfoliation syndrome in cognitive impairment of cerebrovascular or Alzheimer’s type. Acta Ophthalmol Scand 2001; 79: 283-285.
25. Bayer TA, Wirths O: Intracellular accumulation of amyloid-Beta – a predictor for synaptic dysfunction and neuron loss in Alzheimer’s disease. Front Aging Neurosci 2010: 2-8.
26. McKinnon SJ: Glaucoma: ocular Alzheimer’s disease? Front Biosci 2003; 8: 1140-1156.
27. McKinnon SJ, Lehman DM, Kerrigan-Baumrind LA et al.: Caspase activation and amyloid precursor protein cleavage in rat ocular hypertension. Invest Ophthalmol Vis Sci 2002; 43: 1077-1087.
28. Kipfer-Kauer A, McKinnon SJ, Frueh BE, Goldblum D: Distribution of amyloid precursor protein and amyloid-beta in ocular hypertensive C57BL/6 mouse eyes. Curr Eye Res 2010; 35: 828-834.
29. Kowall NW, McKee AC, Yankner BA, Beal MF: In vivo neurotoxicity of beta-amyloid [beta (1-40)] and the beta (25-35) fragment. Neurobiol Aging 1992; 13: 537-542.
30. Tsuruma K, Tanaka Y, Shimazawa M, Hara H: Induction of amyloid precursor protein by the neurotoxic peptide, amyloid-beta 25-35, causes retinal ganglion cell death. J Neurochem 2010; 113: 1545-1554.
31. Guo L, Salt TE, Luong V et al.: Targeting amyloid-beta in glaucoma treatment. Proc Natl Acad Sci USA 2007; 104: 13444-13449.
32. Mohandas E, Rajmohan V, Raghunath B: Neurobiology of Alzheimer’s disease. Indian J Psychiatry 2009; 51: 55-61.
33. Gupta N, Fong J, Ang LC, Yücel YH: Retinal tau pathology in human glaucomas. Can J Ophthalmol 2008; 43: 53-60.
34. Neugroschl J, Sano M: Current treatment and recent clinical research in Alzheimer’s disease. Mt Sinai J Med 2010; 77: 3-16.
35. Jinwal UK, Koren J, O’Leary JC et al.: Hsp70 ATP-ase Modulators as Therapeutics for Alzheimer’s and other Neurodegenerative Diseases. Mol Cell Pharmacol 2010; 2: 43-46.
36. Guo L, Salt TE, Maass A et al.: Assessment of neuroprotective effects of glutamate modulation on glaucoma-related retinal ganglion cell apoptosis in vivo. Invest Ophthalmol Vis Sci 2006; 47: 626-633.
37. Manabe S, Gu Z, Lipton SA: Activation of matrix metalloproteinase-9 via neuronal nitric oxide synthase contributes to NMDA--induced retinal ganglion cell death. Invest Ophthalmol Vis Sci 2005; 46: 4747-4753.
38. Rauen T: Diversity of glutamate transporter expression and function in the mammalian retina. Amino Acids 2000; 19: 53-62.
39. Harada T, Harada C, Nakamura K et al.: The potential role of glutamate transporters in the pathogenesis of normal tension glaucoma. J Clin Invest 2007; 117: 1763-1770.
40. Zoia CP, Tagliabue E, Isella V et al.: Fibroblast glutamate transport in aging and in AD: correlations with disease severity. Neurobiol Aging 2005; 26: 825-832.
41. Lipton SA: Failures and successes of NMDA receptor antagonists: molecular basis for the use of open-channel blockers like memantine in the treatment of acute and chronic neurologic insults. NeuroRx 2004; 1: 101-110.
42. Osborne NN: Recent clinical findings with memantine should not mean that the idea of neuroprotection in glaucoma is abandoned. Acta Opthalmol 2009; 87: 450-454.
43. Li JB, Lu ZG, Xu L et al.: Neuroprotective effects of bis(7)-tacrine against glutamate-induced retinal ganglion cells damage. BMC Neurosci 2010; 6: 11-31.
44. Alvarez R, Alvarez V, Lahoz CH et al.: Angiotensin converting enzyme and endothelial nitric oxide synthase DNA polymorphisms and late onset Alzheimer’s disease. J Neurol Neurosurg Psychiatry 1999; 67: 733-736.
45. Chung KK, David KK: Emerging roles of nitric oxide in neurodegeneration. Nitric Oxide 2010; 22: 290-295.
46. Tsai DC, Hsu WM, Chou CK et al.: Significant variation of the elevated nitric oxide levels in aqueous humor from patients with different types of glaucoma. Ophthalmologica 2002; 216: 346-350.
47. Zanón-Moreno V, Pons S, Gallego-Pinazo R et al.: Involvement of nitric oxide and other molecules with redox potential in primary open angle glaucoma. Arch Soc Esp Oftalmol 2008; 83: 365-372.
48. Kosior-Jarecka E, Gerkowicz M, Latalska M et al.: Nitric oxide level in aqueous humor in patients with glaucoma. Klin Oczna 2004; 106: 158-159.
49. Doganay S, Evereklioglu C, Turkoz Y, Er H: Decreased nitric oxide production in primary open-angle glaucoma. Eur J Ophthalmol 2002; 12: 44-48.
50. Neufeld AH, Sawada A, Becker B: Inhibition of nitric-oxide synthase 2 by aminoguanidine provides neuroprotection of retinal ganglion cells in a rat model of chronic glaucoma. Proc Natl Acad Sci USA 1999; 96: 9944-9948.
51. Moore D, Harris A, Wudunn D et al.: Dysfunctional regulation of ocular blood flow: A risk factor for glaucoma? Clin Ophthalmol 2008; 2: 849-861.
52. Dickstein DL, Walsh J, Brautigam H et al.: Role of Vascular Risk Factors and Vascular Dysfunction in Alzheimer’s Disease. Mt Sinai J Med 2010; 77: 82-102.
53. Moss AM, Harris A, Siesky B et al.: Update and critical appraisal of combined timolol and carbonic anhydrase inhibitors and the effect on ocular blood flow in glaucoma patients. Clin Ophthalmol 2010; 4: 233-241.
54. Likitjaroen Y, Suwanwela NC, Phanthumchinda K: Vasoreactivity induced by acetazolamide in patients with vascular dementia versus Alzheimer’s disease. J Neurol Sci 2009; 283: 32-35.
55. Sun MK, Alkon DL: Carbonic anhydrase gating of attention: memory therapy and enhancement. Trends Pharmacol Sci 2002; 23: 83-89.
56. Supuran CT: Carbonic anhydrases: novel therapeutic applications for inhibitors and activators. Nat Rev Drug Discov 2008; 7: 168-181.
57. He Y, Leung KW, Zhang YH et al.: Mitochondrial complex I defect induces ROS release and degeneration in trabecular meshwork cells of POAG patients: protection by antioxidants. Invest Ophthalmol Vis Sci 2008; 49: 1447-1458.
58. Tezel G: Oxidative stress in glaucomatous neurodegeneration: mechanisms and consequences. Prog Retin Eye Res 2006; 25: 490-513.
59. Mozaffarieh M, Grieshaber MC, Flammer J: Oxygen and blood flow: players in the pathogenesis of glaucoma. Mol Vis 2008; 14: 224-233.
60. Guglielmotto M, Giliberto L, Tamagno E, Tabaton M: Oxidative stress mediates the pathogenic effect of different Alzheimer’s disease risk factors. Front Aging Neurosci 2010; 2: 3.
61. Mozaffarieh M, Grieshaber MC, Orgül S, Flammer J: The potential value of natural antioxidative treatment in glaucoma. Surv Ophthalmol 2008; 53: 479-505.
62. Engin KN: Alpha-tocopherol: looking beyond an antioxidant. Mol Vis 2009; 15: 855-860.
63. Babizhayev MA, Kasus-Jacobi A: State of the art clinical efficacy and safety evaluation of N-acetylcarnosine dipeptide ophthalmic prodrug. Principles for the delivery, self-bioactivation, molecular targets and interaction with a highly evolved histidyl-hydrazide structure in the treatment and therapeutic management of a group of sight-threatening eye diseases. Curr Clin Pharmacol 2009; 4: 4-37.
64. Pescosolido N, Imperatrice B, Karavitis P: The aging eye and the role of L-carnitine and its derivatives. Drugs R D 2008; 9: 3-14.
65. Berdahl JP, Allingham RR, Johnson DH: Cerebrospinal fluid pressure is decreased in primary open-angle glaucoma. Ophthalmology 2008; 115: 763-768.
66. Wostyn P, Audenaert K, De Deyn PP: More advanced Alzheimer’s disease may be associated with a decrease in cerebrospinal fluid pressure. Cerebrospinal Fluid Res 2009, 6: 14.
67. Johnson EC, Guo Y, Cepurna WO, Morrison JC: Neurotrophin roles in retinal ganglion cell survival: lessons from rat glaucoma models. Exp Eye Res 2009; 88: 808-815.
68. Schindowski K, Belarbi K, Buée L: Neurotrophic factors in Alzheimer’s disease: role of axonal transport. Genes Brain Behav 2008; 7: 43-56.
69. Glass CK, Saijo K, Winner B et al.: Mechanisms underlying inflammation in neurodegeneration. Cell 2010; 140: 918-934.
70. Amor S, Puentes F, Baker D, van der Valk P: Inflammation in neurodegenerative diseases. Immunology 2010; 129: 154-169.
71. Tezel G, Wax MB: Glaucoma. Chem Immunol Allergy 2007; 92: 221-227.
72. Farooq MU, Bhatt A: Helicobacter pylori: Neurological and Ophthalmological Disorders. The Internet Journal of Neurology 2008; 9: 2.
73. Drzezga A, Grimmer T, Henriksen G et al.: Effect of APOE genotype on amyloid plaque load and gray matter volume in Alzheimer disease. Neurology 2009; 72: 1487-1494.
74. Sehi M, Grewal DS, Feuer WJ, Greenfield DS: The impact of intraocular pressure reduction on retinal ganglion cell function measured using pattern electroretinogram in eyes receiving latanoprost 0.005% versus placebo. Vision Res 2011; 51: 235-242.