*Małgorzata Staszczyk1, Dorota Kościelniak1, Wirginia Krzyściak2, Małgorzata Jamka-Kasprzyk1, Iwona Kołodziej1, Magdalena Kępisty1, Marcin Magacz2, Anna Jurczak1
In vitro cariogenic model of biofilm formation on selected dental materials – pilot study
Model in vitro tworzenia próchnicotwórczego biofilmu na wybranych materiałach stomatologicznych ? doniesienie wstępne
1Department of Pediatric Dentistry, Institute of Dentistry, Jagiellonian University Medical College, Krakow, Poland
Zakład Stomatologii Dziecięcej, Instytut Stomatologii, Uniwersytet Jagielloński Collegium Medicum, Kraków, Polska
Head of Department: Anna Jurczak, MD, PhD
Kierownik Zakładu: dr hab. n. med. Anna Jurczak
2Department of Medical Diagnostics, Faculty of Pharmacy, Jagiellonian University Medical College, Krakow, Poland
Zakład Diagnostyki Medycznej, Wydział Farmaceutyczny, Uniwersytet Jagielloński Collegium Medicum, Kraków, Polska
actg Head of Department: Wirginia Krzyściak, MD, PhD
p.o. Kierownika Jednostki: dr hab. n. med. Wirginia Krzyściak
Streszczenie
Wstęp. Pomimo postępu w technologii materiałów odtwórczych próchnica wtórna nadal pozostaje problemem.
Cel pracy. Określenie wpływu badanego materiału wypełnieniowego i jego szorstkości na powstawanie próchnicotwórczego biofilmu. Zbadano właściwości nanoceramicznej żywicy kompozytowej i szklano-hybrydowego systemu odbudowy.
Materiał i metody. Ze szczepów Streptococcus mutans i Actinomyces viscosus wyizolowanych z płytki nazębnej dzieci chorych na próchnicę wytworzono biofilmy kariogenne na powierzchni materiałów wypełnieniowych. W niniejszym badaniu określono chropowatość materiału, całkowitą biomasę biofilmu, liczbę żywych komórek w biofilmie oraz mikromorfologię.
Wyniki. Wykazano, że silniejsze wytwarzanie biofilmu jest powiązane z rodzajem stomatologicznych materiałów odtwórczych. Stwierdzono istotne zmniejszenie tworzenia biomasy biofilmu S. mutans i liczby żywych komórek przy zastosowaniu glasjonomeru w porównaniu z kompozytem (p < 0,05) (średnia ± SD: 0,114 ± 0,009 vs 0,076 ± 0,011 po 12 godz.; 0,138 ± 0,006 vs 0,080 ± 0,005 po 72 godz. i średnia ± SD: 1,27 ± 0,09 vs 1,32 ± 0,05 po 12 godz.; 1,28 ± 0,05 vs 1,70 ± 0,07 po 72 godz.). Stwierdzono istotny wpływ zmniejszenia liczby żywych mikroorganizmów A. viscosus w biofilmie na materiale glasjonomerowym w porównaniu z kompozytem (średnia ± SD: 1,10 ± 0,05 vs 1,20 ± 0,05 po 12 godz.; 1,11 ± 0,05 vs. 1,30 ± 0,05 po 72 godz.). Całkowita biomasa biofilmu wytworzona przez A. viscosus na materiale kompozytowym była niższa w porównaniu z grupą kontrolną (średnia ± SD: 0,122 ± 0,011 vs. 0,132 ± 0,006 po 12 godz.; 0,132 ± 0,007 vs. 0,161 ± 0,007 po 72 godz.), ale wartości gęstości optycznej (OD) wytworzonej biomasy biofilmu wskazują na jej wyraźniejsze działanie hamujące na biofilm A. viscosus w porównaniu z kompozytem. Zaobserwowano istotne hamowanie tworzenia biofilmu A. viscosus na glasjonomerach w porównaniu z grupą kontrolną (średnia ± SD: 0,122 ± 0,004 vs. 0,132 ± 0,006 po 12 godz.; 0,122 ± 0,005 vs. 0,161 ± 0,007 po 72 godz.).
Wnioski. Lepsze właściwości przeciw biofilmowi glasjonomeru w porównaniu z materiałem kompozytowym mogą potwierdzać przewagę materiałów glasjonomerowych w profilaktyce próchnicy wtórnej oraz w publicznej opiece stomatologicznej dzieci.
Summary
Introduction. Despite the progress made in restorative materials technology, secondary caries still remains a problem.
Aim. Present research aimed to investigate how the selected types of material and their roughness affected cariogenic biofilm formation. Nano-ceramic composite resin and glass-hybrid restorative system properties have been investigated.
Material and methods. Clinical strains of Streptococcus mutans and Actinomyces viscosus were isolated from plaque of children with caries and the cariogenic biofilm was formed on materials surface. In present study material roughness, total biofilm biomass, number of viable cells in biofilm and micromorphology was determined.
Results. Stronger biofilm generation has been shown to be associated with the type of dental restorative materials. A significant reduction was found in the S. mutans biofilm biomass formation and number of viable cells under the influence of application of glass ionomer compared to composite (p < 0.05) (mean ± SD: 0.114 ± 0.009 vs 0.076 ± 0.011 after 12 h; 0.138 ± 0.006 vs. 0.080 ± 0.005 after 72 h and mean ± SD: 1.27 ± 0.09 vs 1.32 ± 0.05 after 12 h; 1.28 ± 0.05 vs. 1.70 ± 0.07 after 72 h). A significant effect of the reduction of the number of A. viscosus viable microorganisms in the biofilm was identified on the glass ionomer material compared to the composite (mean ± SD: 1.10 ± 0.05 vs 1.20 ± 0.05 after 12 h; 1.11 ± 0.05 vs. 1.30 ± 0.05 after 72 h). The total biofilm biomass formed by A. viscosus on the composite material was lower compared to the controls (mean ± SD: 0.122 ± 0.011 vs. 0.132 ± 0.006 after 12 h; 0.132 ± 0.007 vs. 0.161 ± 0.007 after 72 h) but the optical density (OD) values of the created biofilm biomass indicate its more pronounced inhibitory effect on A. viscosus biofilm, as compared to the composite. Significant inhibitions of A. viscosus biofilm formation on glass ionomers have been observed compared to the controls (mean ± SD: 0.122 ± 0.004 vs. 0.132 ± 0.006 after 12 h; 0.122 ± 0.005 vs. 0.161 ± 0.007 after 72 h).
Conclusions. Better anti-biofilm properties of glass ionomer compared with composite material can prove the advantage of glass ionomer materials in the prevention of secondary caries and in community children dental care.
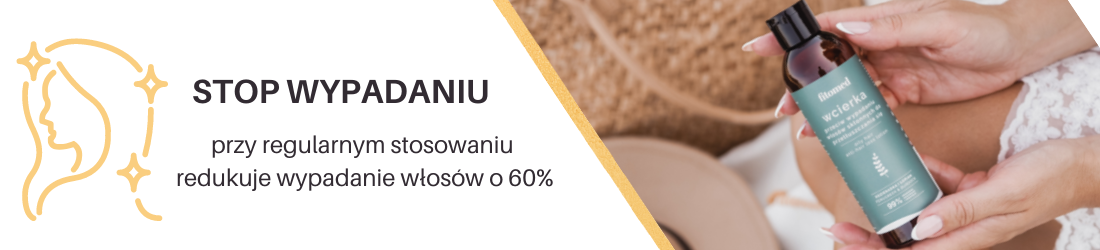
Introduction
Secondary caries seems to be a serious problem in children, especially in the early childhood. Treatment of this process generates huge costs and untreated may lead to many complications, even severe, local and general in the child’s organism. Dental materials become more and more modern and durable, but problem remains.
Contemporary dentistry places particular emphasis on caries prevention and minimally invasive treatment, saving hard tooth tissues, which should be the procedure of choice in each case of restorative treatment, using adhesive materials that meet strength and aesthetic requirements while maintaining proper indications for their use (1).
Each decision regarding the use of a specific restorative material must be individualized and be based on knowledge of the composition, physico-chemical properties and characteristics of the restorative material. When choosing the material, factors related to the severity of the carious lesion, the ability to control moisture during the procedure, oral hygiene and patient preferences should be taken into account. All this is aimed at reducing the development of secondary caries, or failure in the form of loss of all or part of the filling.
The problem of choosing restorative materials is also often associated with the economic possibilities of patients, and in a broader sense ? with the economic model of organized healthcare in each country.
Composite materials, currently considered as basic restorative materials for reconstruction of anterior and posterior teeth, are recommended for permanent teeth fillings in all cavity classes (2). However, according to the results of the trials, currently the most commonly used materials for the reconstruction of cavities I and II class according to Black in deciduous molars are resin-modified glass-ionomer cements having at the same time remineralizing properties (3, 4). The lower popularity of fillings made of composite materials in primary dentition than in permanent dentition can be explained by the relatively complicated procedure of application of these materials, its multi-stage can be an important factor reducing the durability of fillings (5).
However, there are many doubts as to which materials are optimal for primary and permanent teeth. The insufficient number of randomized clinical trials available in the literature comparing various materials used to fill defects, especially in primary teeth, means that there is insufficient evidence to formulate clear clinical recommendations (6).
Nevertheless, secondary caries remains a big problem regardless of technological progress related to the quality of these materials (7, 8). The emerging dysbiosis between the cariogenic microorganisms species producing acids and the physiological flora of the host has an impact on their mechanical properties and is often the cause of micro-leaks of used dental materials, facilitating recurrent dental damage (9, 10). It is the main reason causing failure of restorations (11).
Streptococcus mutans is associated with the initiation of caries and is also isolated both from enamel carious lesions and hidden/closed dentinal lesions/dentin caries (12). S. mutans could be found in healthy and diseased sites and suggested that other species of Lactobacillus, Prevotella, Propionibacterium, nonmutans streptococci and Actinomyces also played important roles in caries initiation and biofilm community interactions (13-15).
Actinomyces viscosus, like Streptococcus mutans, is one of the most common supragingival plaque-forming cariogenic microorganisms (16-18). In addition to Lactobacillus casei, as strongly acidogenic and aciduric, it is also one of the two predominant deep caries microorganisms and one of the main etiological factors of secondary dental caries (19-22). Regulation of adhesive ability to the tooth surface of the above microorganisms can therefore be a good strategy for controlling pathogenic biofilms by affecting the relationship between the dental material used for the fillings and oral microflora, thus reducing or delaying the occurrence of secondary carious lesions in the teeth (23, 24).
Adhesive properties of the above microorganisms are the key features determining their pathogenicity, while their interactions with dental materials and the rest of the oral microflora may determine the activity of these features and the bacterial virulence under favourable conditions (9, 25, 26). Experimental data showed that the surface of the dental material, interaction of microorganisms and nutrient substrates needed for the metabolism of these bacteria determine the development or absence of cariogenic biofilm and secondary lesions.
Aim
The aim of the study was to assess and compare cariogenic biofilm formation on two dental materials in in vitro models with the use of clinical wild strains of S. mutans and A. viscosus isolated from children with caries.
Material and methods
Study groups
The study was performed in accordance with the Helsinki Declaration of 2013. Informing consent to the study procedure was obtained from all participants. The protocol was approved by the Bioethics Committee at the Jagiellonian University in Cracow (No. 1072.6120.183.2017).
The study was conducted between 2019 and 2021 and included 40 pediatric patients aged between 4-9 years old from Department of Pediatric Dentistry, Jagiellonian University Dental Clinic in Cracow, Poland. The study included bacterial strains isolated from dental plaque and saliva of patients diagnosed with caries. The results marked with reference numbers were entered onto a standardized examination chart. Examinations were conducted based on the criteria established by the World Health Organization for epidemiological studies (27).
The research group consisted of children with caries in dentin, defined as decay group 4-6 in the ICDAS II codes (International Caries Detection and Assessment System Coordinating Committee) (28).
The exclusion criteria were as follows: age below 4 or above 9 years, diabetes, periodontal disease, epithelial dysplasia, and inflammatory lesions of the oral mucosa. Antibiotics, non-steroid, anti-inflammatory medications, corticosteroids, and vitamin intake as well as professional fluoride prophylaxis within the last 3 months also resulted in a patient’s exclusion from the study. Dental plaque was determined using the OHI-S index (Simplified Oral Hygiene Index) (29).
Preparation of the samples
A composite nano-ceramic filler Ceram X® (One Universal, Dentsply, DeTrey, Konstanz, Germany) and a glass-hybrid glass ionomer for bulk-fil-type fillings (EQUIA® Forte Fil, GC, Tokyo, Japan) were used in the study. These two materials were the most used from glass ionomers and composites group in the Department of Pediatric Dentistry for dental fillings in children. 40 samples were prepared according to the manufacturer’s instructions for each of the materials. Ceram X® material samples with a thickness not exceeding 2 mm were irradiated with a 1200 mW/cm2 polymerization diode lamp (GC D-Light Duo) for 20 seconds. Before preparing the samples from the glass ionomer, the capsule with the material was placed in a shaker and mixed for 10 sec. After the recommended setting time of 2.5 min, samples were covered with EQUIA® Forte Coat varnish and cured with light using a diode polymerization lamp for 20 sec (30).
The prepared materials were stored under lightproof and microaerophilic conditions prevailing in the mouth (laboratory incubator: 37°C, atmosphere enriched with 10% CO2, > 95% relative humidity) for 24 h to allow complete polymerization of the composite and the glass ionomer. The slides were sequentially sterilized by the plasma sterilizer for one hour at 45°C to fix the resin surface.
In order to assess the homogeneity of the surface of the tested materials, their roughness was measured (SR, n = 10 of each material) using a needle profilometer (Bruker DektakXT, Germany). 10 randomly selected 1.75 mm fragments were measured in three-line scans for each material using a diamond tip with a radius of 2 μm and a tip angle of 90°. The cut-off level was set to 0.25. Data were expressed as Ra (μm).
The materials fixed on the slides were then transferred to the sterile microtiter plates containing 2 ml sterile PBS (Phosphate Buffered Saline) in each well and stored at a room temperature for another 10 days to allow unreacted monomers to leach from the slides coated with dental materials.
Saliva and dental plaque collecting
Saliva samples were collected from all participants according to the protocol proposed by Szczeklik et al., 2 hours after morning tooth brushing with fluoride toothpaste (31). The participating subjects were fasting before saliva collection. Participants rinsed they mouth with deionised water for 30 sec. and expectorated oral contents. The stimulated saliva was collected by chewing cotton swab for 3 min. Soaked swabs were placed in salivettes which were immediately centrifuged for 2 min at 4°C and 1000 rpm. Collected material was stablished to sterile 1.5 ml Eppendorf type tubes which were frozen at -80°C until assayed. Dental plaque from all tooth surfaces was collected using sterile dental brush according to the protocol proposed by Krzyściak et al. (32).
Microbiological analysis
The samples were transported within 4 hours to the laboratory in 1 ml sterile physiological saline, pH 7.0 (PBS) at room temperature. The samples were disrupted by gentle vortexing and sonication for 30 sec. Subsequently, serial dilutions of the starting solution were prepared in sterile physiological saline. Samples with the above dilutions were inoculated on plates with 10% blood agar and on selective media used to detect certain microorganism groups described by Krzyściak et al. (32).
The inoculated media were incubated in microaerophilic conditions in the presence of 5% CO2 at a temperature of 37°C over a period of 24-48 hours. Based on their morphology, grown on selective media were counted.
The bacteria species obtained in the tests were verified by means of mass spectrometry (MS) system MALDI with TOF (Bruker Daltonik, Germany). The identification of bacteria species was possible by comparison of obtained peptides molecular weight, charge and time-of-flight distribution spectra with reference spectra from database (MALDI Biotyper 3.0 software). The likehood of correct identification was expressed as point indicator. The value of that indicator for reliable identification in this study was stablished as 2.000 or greater.
Biofilm model
Microbiological tests with 40 clinical isolated S. mutans and A. viscosus strains were carried out in 24-well sterile flat-bottomed microtiter plates with the covered polystyrene slides of a 12 mm diameter placed on the bottom.
Prepared samples of composite materials and glass ionomer were placed in 2.0 ml of BHI culture medium with 5% sucrose containing bacterial strains and incubated for 72 h at 37°C in microaerophilic conditions in a 10% CO2 atmosphere. Inoculation of the medium with a suspension of pure cultures was carried out in a logarithmic phase of bacterial growth at a concentration of 1 × 107 cells/ml.
The control samples (polystyrene disks without dental material) contained bacterial suspensions of S. mutans and A. viscosus reference strains: ATCC 25175 and ATCC 43146 in 0.9% NaCl which were incubated under the same conditions as the test samples.
In vitro biofilm tests
The ability of biofilm formation on the selected dental materials was assessed using a closed model based on a microtiter plate. The formed biofilm biomass was measured by crystal violet (CV) staining according to the method described in our previous studies (33).
100 μl of the standardized suspension of S. mutans and A. viscosus was transferred to each well of a 96-well microtiter plate coated with the tested dental materials, which was then incubated for 90 min at 37°C to initiate an adhesion of the tested bacterial strains. The suspension was aspirated and each well was washed three times with PBS to remove planktonic cells. 100 μl of fetal bovine serum was consequently added to each well and incubated under the same conditions for 8 h. The wells were washed three times with PBS and 100 μl of a standardized bacterial suspension was added to each well. Control samples contained 100 μl PBS (PBS control) or 100 ml BHI broth with 5% sucrose. The medium was changed once and the plates were incubated for 72 h.
Biofilm generation by CFU/ml determination
To determine the number of bacteria in the biofilm, the cultures were performed after thoroughly rinsing the plates with sterile PBS, scraping the biofilm from the bottom of the wells and suspending it in 100 μl sterile saline. Such a bacterial suspension was serially diluted and inoculated on BHI agar media with 5% sheep blood. The number of grown colonies was counted after 24, 48 and 72 h of overnight culture and expressed as CFU/ml according to the formula:
CFU/ml = (number of colonies on the plate × 10) ÷ dilution.
Analysis of biofilm formation by determining its total biomass
Biofilm biomass was assessed after 24, 48 and 72 h of incubation by staining with CV. The biofilm was dipped in 99% methanol for 20 min and then air dried. 125 μl of 0.1% CV solution was added to the wells which were washed three times with PBS after 20 min. The plates were dried by shaking after each washing step. After the final washing step, the samples were allowed to dry completely. Finally, the bound CV was released by adding 200 μl of 95% ethanol followed by incubation for 15 min at a room temperature. The contents of the wells were mixed by repeated pipetting, and then 125 μl of the suspension was transferred to the wells of a clean 96-well flat-bottom microtiter plate. Absorbance was measured at a wavelength of 540 nm. All steps were carried out at a room temperature. The study was conducted in two independent experiments, separately for S. mutans and A. viscosus. The biofilm formation curve was plotted based on the obtained data.
Analysis of biofilm formation using scanning electron microscopy (SEM)
Powyżej zamieściliśmy fragment artykułu, do którego możesz uzyskać pełny dostęp.
Mam kod dostępu
- Aby uzyskać płatny dostęp do pełnej treści powyższego artykułu albo wszystkich artykułów (w zależności od wybranej opcji), należy wprowadzić kod.
- Wprowadzając kod, akceptują Państwo treść Regulaminu oraz potwierdzają zapoznanie się z nim.
- Aby kupić kod proszę skorzystać z jednej z poniższych opcji.
Opcja #1
29 zł
Wybieram
- dostęp do tego artykułu
- dostęp na 7 dni
uzyskany kod musi być wprowadzony na stronie artykułu, do którego został wykupiony
Opcja #2
69 zł
Wybieram
- dostęp do tego i pozostałych ponad 7000 artykułów
- dostęp na 30 dni
- najpopularniejsza opcja
Opcja #3
129 zł
Wybieram
- dostęp do tego i pozostałych ponad 7000 artykułów
- dostęp na 90 dni
- oszczędzasz 78 zł
Piśmiennictwo
1. Banerjee A, Frencken JE, Schwendicke F, Innes NPT: Contemporary operative caries management: Consensus recommendations on minimally invasive caries removal. Br Dent J 2017; 223(3): 215-222.
2. Demarco FF, Collares K, Correa MB et al.: Should my composite restorations last forever? Why are they failing? Braz Oral Res 2017; 31: 92-99.
3. Nicholson JW, Sidhu SK, Czarnecka B: Fluoride exchange by glass-ionomer dental cements and its clinical effects: a review. Biomater Investig Dent 2023; 10(1): 5-8.
4. Kosior P, Klimas S, Nikodem A et al.: An in vitro examination of fluoride ions release from selected materials ? resin-modified glass-ionomer cement (Vitremer) and nanohybrid composite material (Tetric EvoCeram). Acta Bioeng Biomech 2023; 25(1): 101-115.
5. Ge KX, Quock R, Chu CH, Yu OY: The preventive effect of glass ionomer cement restorations on secondary caries formation: A systematic review and meta-analysis. Dent Mater 2023; 39(12): 1-17.
6. Weldon JC, Yengopal V, Siegfried N et al.: Dental filling materials for managing carious lesions in the primary dentition. Cochrane Database of Syst Rev 2016; 2016 (9).
7. Aung YM, Tin Tin S, Jelleyman T, Ameratunga S: Dental caries and previous hospitalisations among preschool children: Findings from a population-based study in New Zealand. N Z Med J 2019; 132(1493): 44-53.
8. dos Santos Letieri A, Freitas-Fernandes LB, Valente APC et al.: Longitudinal evaluation of salivary IgA-S in children with early childhood caries before and after restorative treatment. J Clin Pediatr Dent 2019; 43(4): 239-243.
9. Bächle, J, Merle C, Hahnel S, Rosentritt M: Bacterial Adhesion on Dental Polymers as a Function of Manufacturing Techniques. Materials 2023; 16(6): 2373.
10. Jassal M, Mittal S, Tewari S: Clinical effectiveness of a resin-modified glass ionomer cement and a mild one-step self-etch adhesive applied actively and passively in noncarious cervical lesions: An 18-month clinical trial. Operative Dent 2018; 43(6): 581-592.
11. Gao SS: The longevity of posterior restorations in primary teeth. Evidence-Based Dent 2018; 19(2): 44.
12. Simón-Soro A, Mira A: Solving the etiology of dental caries. Trends Microbiol 2015; 23(2): 76-82.
13. Simón-Soro A, Guillen-Navarro M, Mira A: Metatranscriptomics reveals overall active bacterial composition in caries lesions. J Oral Microbiol 2014; 6: 25443.
14. Aas JA, Griffen AL, Dardis SR et al.: Bacteria of dental caries in primary and permanent teeth in children and young adults. J Clin Microbiol 2008; 46(4): 1407-1417.
15. Ribeiro A, Azcarate-Peril MA, Cadenas MB et al.: The oral bacterial microbiome of occlusal surfaces in children and its association with diet and caries. PloSOne 2017; 12(7): 1-16.
16. Tu Y, Deng S, Wang Y et al.: Adhesive ability of different oral pathogens to various dental materials: an in vitro study. Can J Infect Dis Med Microbiol 2022; 1: 5-8.
17. Farva K, Sattar H, Ullah H et al.: Phenotypic analysis, molecular characterization, and antibiogram of caries-causing bacteria isolated from dental patients. Microorganisms 2023; 11(8): 17-21.
18. Gunay A, Celenk S, Adiguzel O et al.: Comparison of antibacterial activity, cytotoxicity and fluoride release of glass ionomer restorative dental cements in dentistry. Med Sci Monit 2023; 23(29): 5-7.
19. Ahrari F, Mazhari F, Ghazvini K et al.: Antimicrobial photodynamic therapy against Lactobacillus casei using curcumin, nano-curcumin, or erythrosine and a dental LED curing device. Lasers Med Sci 2023; 38(1): 260.
20. Wu J, Jiang X, Yang Q et al.: Inhibition of Streptococcus mutans biofilm formation by the joint action of oxyresveratrol and Lactobacillus casei. Appl Environ Microbiol 2022; 88(9): 6-9.
21. Metz I, Rothmaier K, Pitchika V et al.: Risk factors for secondary caries in direct composite restorations in primary teeth. Int J Paediatr Dent 2015; 25(6): 451-461.
22. Pørksen CJ, Keller MK, Damholt A et al.: The effect of a lozenge combining prebiotic arginine and probiotics on caries increment in children during 10-12 months, a randomized clinical trial. J Dent 2023; 135: 6-9.
23. Krzyściak W, Jurczak A, Kościelniak D et al.: The virulence of Streptococcus mutans and the ability to form biofilms. Eur J Clin Microbiol Infect Dis 2014; 33(4): 499-515.
24. Tawakoli PN, Attin T, Mohn D: Oral biofilm and caries-infiltrant interactions on enamel. J Dent 2016; 48: 40-45.
25. Nedeljkovic I, de Munck J, Vanloy A et al.: Secondary caries: prevalence, characteristics, and approach. Clin Oral Investig 2020; 24(2): 683-691.
26. Tu Y, Ren H, He Y et al.: Interaction between microorganisms and dental material surfaces: general concepts and research progress. Journal of oral microbiology 2023; 1: 15-19.
27. WHO: Oral Health Surveys: Basic Methods, 5th ed.; WHO: Geneva, Switzerland, 2013.
28. Banting DW, Eggertsson H, Zandoná, AGF et al.: Rationale and Evidence for the International Caries Detection and Assessment System (ICDAS II). International Caries Detection and Assessment System Coordinating Committee 2012 https://www.iccms-web.com/uploads/asset/592848be55d87564970232.pdf.
29. Wei SH, Lang NP: Periodontal epidemiological indices for children and adolescents: II. Evaluation of oral hygiene; III. Clinical applications. Pediatr Dent 1982: 4(1): 64-73.
30. Kleverlaan CJ, van Duinen RNB, Feilzer AJ: Mechanical properties of glass ionomer cements affected by curing methods. Dental Materials 2004; 20(1): 45-50.
31. Szczeklik K, Owczarek D, Cibor D et al.: Relative homogeneity of oral bacterial oral in Crohn’s disease compared to ulcerative colitis and its connections with antioxidant defense ? preliminary report. Folia Med Cracov 2019; 59(1): 15-35.
32. Krzyściak W, Papież M, Jurczak A et al.: Relationship between pyruvate kinase activity and cariogenic biofilm formation in Streptococcus mutans biotypes in caries patients. Front Microbiol 2017; 8(5): 856.
33. Krzyściak W, Kościelniak D, Papież M et al.: Effect of a Lactobacillus Salivarius Probiotic on a Double-Species Streptococcus Mutans and Candida Albicans Caries Biofilm. Nutrients 2017; 9(11): 1242.
34. R Development Core Team 3.0.1: A Language and Environment for Statistical Computing. R Foundation for Statistical Computing. R Foundation for Statistical Computing; 2013. Available from: http://www.r-project.org.
35. Valero-Mora PM: ggplot2: Elegant Graphics for Data Analysis. Journal of Statistical Software. 2nd ed. Springer; 2010.
36. Yulianto HDK, Rinastiti M, Cune MS et al.: Biofilm composition and composite degradation during intra-oral wear. Dent Mater 2019; 35(5): 740-750.
37. Kreth J, Merritt J, Pfeifer CS et al.: Interaction between the oral microbiome and dental composite biomaterials: where we are and where we should go. J Dent Res 2020; 99(10): 1140-1490.
38. Engel AS, Kranz HT, Schneider M et al.: Biofilm formation on different dental restorative materials in the oral cavity. BMC Oral Health 2020; 20(1): 162.
39. Lin NJ, Keeler C, Kraigsley AM et al.: Effect of dental monomers and initiators on Streptococcus mutans oral biofilms. Dent Mater 2018; 34(5): 776-785.
40. Hahnel S, Ionescu AC, Cazzaniga G et al.: Biofilm formation and release of fluoride from dental restorative materials in relation to their surface properties. J Dent 2017; 60: 14-24.
41. Krämer N, Schmidt M, Lücker S et al.: Glass ionomer cement inhibits secondary caries in an in vitro biofilm model. Clin Oral Invest 2018; 22(2): 1019-1031.
42. Amend S, Frankenberger R, Lücker S et al.: Secondary caries formation with a two-species biofilm artificial mouth. Dent Mater 2018; 34(5): 786-796.
43. Cazzaniga G, Ottobelli M, Ionescu AC et al.: In vitro biofilm formation on resin-based composites after different finishing and polishing procedures. J Dent 2017; 67: 43-52.
44. Barbosa RPDS, Pereira-Cenci T, Silva WM et al.: Effect of cariogenic biofilm challenge on the surface hardness of direct restorative materials in situ. J Dent 2012; 40(5): 359-363.
45. Du X, Huang X, Huang C et al.: Inhibition of early biofilm formation by glass-ionomer incorporated with chlorhexidine in vivo: A pilot study. Aust Dent J 2012; 57(1): 58-64.
46. Tomiyama K, Ishizawa M, Watanabe K et al.: Antibacterial effects of surface pre-reacted glass-ionomer (S-PRG) filler eluate on polymicrobial biofilms. Am J Dent 2023; 36(2): 91-94.
47. Dhananjaya KM, Chakraborty M, Vadavadagi SV et al.: A scanning electron microscope evaluation of the efficacy of different fluoride-releasing dental restorative materials to prevent enamel demineralization: an in vitro study. J Contemp Dent Pract 2021; 22(11): 1292-1296.
48. Borges FT, Campos WR da C, Munari LS ana et al.: Cariostatic effect of fluoride-containing restorative materials associated with fluoride gels on root dentin. J Appl Oral Sci 2010; 18(5): 453-460.
49. Selwitz RH, Ismail AI, Pitts NB: Dental caries. Lancet 2007; 369(9555): 51-59.
50. Eick S, Glockmann E, Brandl B, Pfister W: Adherence of Streptococcus mutans to various restorative materials in a continuous flow system. J Oral Rehab 2004; 31(3): 278-285.
51. de Faria NS, Moura LKB, de Macedo LMD et al.: Effect of a 12-methacryloyloxy-dodecyl-pyridinium-bromide-containing adhesive with different post types on the long-term bond strength to dentin. European J Oral Sci 2017; 125(5): 403-409.
52. Zhang Y, Chen Y, Hu Y et al.: Quaternary ammonium compounds in dental restorative materials. Dent Mater J 2018; 37(2): 183-191.
53. Poornima P, Koley P, Kenchappa M, et al.: Comparative evaluation of compressive strength and surface microhardness of EQUIA Forte, resin-modified glass-ionomer cement with conventional glass-ionomer cement. J Indian Soc Pedod Prev Dent 2019; 37(3): 265-270.
54. Nicholson JW, Sidhu SK, Czarnecka B: Enhancing the mechanical properties of glass-ionomer dental cements: a review. Materials (Basel) 2020; 13(11): 2510.
55. Qvist V, Poulsen A, Teglers PT, Mjör IA: The longevity of different restorations in primary teeth. Int J Paediatr Dent 2010; 20(1): 1-7.