© Borgis - Postępy Nauk Medycznych 1/2012, s. 7-11
*Magdalena Kuźma-Kozakiewicz, Hubert Kwieciński
Terapia genowa stwardnienia bocznego zanikowego
Gene therapy of amyotrophic lateral sclerosis**
Katedra i Klinika Neurologii, Warszawski Uniwersytet Medyczny
Kierownik Katedry i Kliniki: prof. dr hab. med. Anna Kamińska
Streszczenie
Stwardnienie boczne zanikowe (SLA) jest śmiertelną chorobą neurozwyrodnieniową dotyczącą niemal 500 000 osób na świecie. Około 35% przypadków rodzinnych i 10% sporadycznych jest spowodowanych mutacjami genu SOD1 (kodującego miedziowo-cynkową dysmutazę ponadtlenkową), FUS/TLS (białko FUS/TLS) oraz TARDP (białko TDP-43). Te grupy chorych mają największą szansę skorzystania z terapii genowej. Artykuł opisuje główne kierunki rozwoju strategii leczniczych opartych na wyciszaniu genów w stwardnieniu bocznym zanikowym.
Summary
Amyotrophic lateral sclerosis (ALS) is a fatal neurodegenerative disorder affecting nearly half a million people worldwide. Approximately 35% of familial and 10% of sporadic cases are due to mutations in genes encoding for CuZn superoxide dismutase (SOD1), transactive response DNA-binding protein TDP-43 (TARDP) and fused in sarcoma/translocated in liposarcoma protein (FUS/TLS). These subpopulations of patients may be the first to profit from gene therapy. We here describe current directions of development of ALS treatment based on gene silencing.
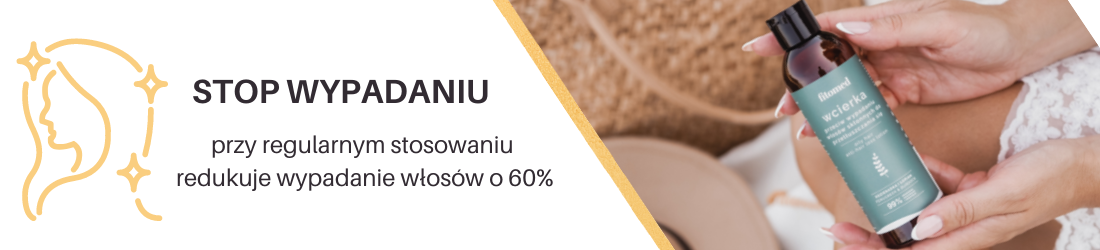
Introduction
Amyotrophic lateral sclerosis (ALS) is a neurodegenerative disorder affecting over 2500 people in Poland and nearly half a million people worldwide. It is sporadic in approximately 90% of cases (sporadic ALS, SALS). The remaining 10-15% of patients suffers from a familial condition mostly inherited as an autosomal dominant trait (familial ALS, FALS). From 12 to 23.5% of FALS and over 7% of SALS cases are due to mutations in a gene encoding for Cu, Zn-superoxide dismutase (SOD1), a free radical scavenging enzyme (1). Several new genes were found to be associated with classic ALS in recent years. They encode for transactive response (TAR) DNA-binding protein TDP-43 (TARDP), fused in sarcoma/translocated in liposarcoma protein (FUS/TLS), each responsible for 4% of FALS and 1% of SALS, angiogenin (ANG), optineurin (OPTN) and others (2, 3). The greatest number of mutations associated with ALS was found in the SOD1 gene (for complete list please refer to http://www.alsod.org, http://alsod1.iop.kcl.ac.uk/reports/mutations). A vast majority of over 155 causative SOD1 mutations identified to date induce changes in stability of the enzyme. Some also influence its enzymatic activity. Experiments with the use of transgenic animals harboring human mutated SOD1 have shown that mutations in the SOD1 gene lead to a toxic gain of function of the SOD1 protein. In transgenic mice, the disease phenotype directly correlates with the level of transgene expression (4). On the other hand, the animals deprived of wild type SOD1 (wt SOD1) do not develop ALS-like phenotype. Overall, the experimental data leads to conclusion that genetic silencing of mutant SOD1 may potentially decrease the mutation-induced toxicity and ameliorate the clinical phenotype (5).
The mutation in TARDP, FUS/TLS, ANG and OPTN associated with FALS, are also inherited in an autosomal dominant manner (with only single exceptions). Both mutated TDP-43 and FUS/TLS form intraneuronal and glial inclusions, what may suggest a toxic gain of protein function rather than its loss (6). In fact, increased expression of TDP-43 in the nuclei was proved toxic to neurons (7). On the other hand, a depletion of TDP-43 from mouse adult brain with antisense oligonucleotides resulted in numerous abnormal splicing events. It also lead to decrease of mRNA levels of several genes involved in synaptic activity, including FUS/TLS and progranulin (8). Silencing of either TARDBP or FUS/TLS by RNA interference was also shown to reduce the expression of histone deacetylase (HDAC) 6 mRNA in cell culture (9). The impact of such silencing on mammal physiology has not been evaluated. The lack of similar experiments designed for other genes, reduces their consideration as immediate candidates for gene therapy. More information on the physiological and pathological function of the newly discovered genes associated with ALS will arrive along with new in vivo studies (2).
For these reasons, at present, the gene therapy mostly orientates on patients who carry ALS-associated mutations in the SOD1 gene. The autosomal dominant inheritance and toxic gain of protein function makes them prone to profit from therapy based on gene silencing. A specific reduction of expression of a target gene would assure the therapeutic effects. Two molecular mechanisms of potential use is gene silencing have been described to date. They are RNA interference and antisense olignucleotides.
RNA interference (RNAi)
RNAi is a highly conserved cellular mechanism responsible for gene silencing. It is triggered by double stranded RNAs (dsRNAs) capable of degrading the complementary mRNA or blocking its translation. RNAi is a natural cell defense against viruses and excessive transposon mobilization. It also regulates gene expression during development (10, 11). DsRNAs may be synthesized within the nucleus as pre-microRNA (miRNA), or introduced to the cell by viral vectors or plasmids. In either case, dsRNAs are processed to small (21-23 nucleotide)-interfering RNAs (siRNAs) by RNase III-like enzyme, called Dicer (12). siRNAs are effector RNAs, which target cognate cellular mRNAs and recruit them to the cytosolic RNA-induced silencing complex (RISC), a multiprotein complex containing ribonuclease. Attachment to RISC leads to an ATP-dependent mRNA unwinding and binding a single stranded RNA to the target mRNA. The process ends by mRNA rapid degradation catalyzed by cellular exonucleases present in the cytoplasm or, dependent on the degree of complementarity, the inhibition of translation (12, 13). MiRNA are non coding RNAs responsible for gene regulation and not the protein synthesis. They are produced by Dicer from endogenous short hairpin RNAs (shRNA) precursors (12). After binding to protein complex they associate to ribosomes thus inhibiting translation of partially compatible mRNA. If miRNA is thoroughly complementary to the target RNA, it produces target degradation, just like in the case of exogenous siRNA. The possibility to use RNAi in gene therapy arouse from a study that permitted to trigger gene silencing with the use of exogenous siRNAs (14). It’s worth noticing that introducing ds RNAs longer than 23 nt provokes enhanced immune response mediated by interferons and inflammatory cytokines, which leads to global repression of translation and cell death (15). Since RNA interference is quite specific, it may be therapeutically used to repress expression of genes responsible for synthesis of mutated proteins with toxic properties (16). In light of our knowledge considering genetic background of ALS, and more particularly on ALSOD (ALS due to SOD1 mutation), this disorder seems to be a perfect candidate for treatment using RNAi-based approach.
The biggest concern about the use of RNAi in therapy is to assure its efficient transport to target areas. siRNAs do not cross the blood-brain barrier and are highly instable in biological fluids. They need to be introduced to the RISC in the cytoplasm by direct CSF/brain parenchyma infusion (anterograde transport). Since they are rapidly degraded after gene silencing (36-48 h), the potential effectiveness of this therapy implicates introduction of numerous siRNA copies. Unexpectedly, even very short siRNAs induce interferon response in a dose-dependent manner (17). The only solution of this issue seems to be a long-term repetitive siRNA administration, which may encounter important technical problems.
Powyżej zamieściliśmy fragment artykułu, do którego możesz uzyskać pełny dostęp.
Mam kod dostępu
- Aby uzyskać płatny dostęp do pełnej treści powyższego artykułu albo wszystkich artykułów (w zależności od wybranej opcji), należy wprowadzić kod.
- Wprowadzając kod, akceptują Państwo treść Regulaminu oraz potwierdzają zapoznanie się z nim.
- Aby kupić kod proszę skorzystać z jednej z poniższych opcji.
Opcja #1
29 zł
Wybieram
- dostęp do tego artykułu
- dostęp na 7 dni
uzyskany kod musi być wprowadzony na stronie artykułu, do którego został wykupiony
Opcja #2
69 zł
Wybieram
- dostęp do tego i pozostałych ponad 7000 artykułów
- dostęp na 30 dni
- najpopularniejsza opcja
Opcja #3
129 zł
Wybieram
- dostęp do tego i pozostałych ponad 7000 artykułów
- dostęp na 90 dni
- oszczędzasz 78 zł
Piśmiennictwo
1. Kuźma-Kozakiewicz M, Kwieciński H: The genetics of amyotrophic lateral sclerosis. Pol Neurol Neurosurg 2009; 43(6): 538-549.
2. Lagier-Tourenne C, Polymenidou M, Cleveland DW: TDP-43 and FUS/TLS: emerging roles in RNA processing and neurodegeneration. Hum Mol Gen 2010; 19(1): R46-R64.
3. van Blitterswijk M, van Vught PW, van Es MA: Novel optineurin mutations in sporadic amyotrophic lateral sclerosis patients. Neurobiol Aging 2011; Jul 27 (epub ahead of print).
4. Gurney ME, Pu H, Chiu AY et al.: Motor neuron degeneration in mice that express a human Cu, Zn superoxide dismutase mutation. Science 1994; 264(5166): 1772-5.
5. Reaume AG, Elliott JL, Hoffman EK et al.: Motor neurons in Cu/Zn superoxide dismutase-deficient mice develop normally but exhibit enhanced cell death after axonal injury. Nat Genet 1996; 13(1): 43-7.
6. Bowers WJ, Breakefield XO, Sena-Esteves M: Genetic therapy for the nervous system. Hum Mol Gen 2011; 20(1): R28-R41.
7. Wang DB, Gitcho MA, Kraemer BC et al.: Genetic strategies to study TDP-43 in rodents and to develop preclinical therapeutics for amyotrophic lateral sclerosis. Eur J Neurosci 2011; Jul 21 (Epub ahead of print).
8. Polymenidou M, Lagier-Tourenne C, Hutt KR: Long pre-mRNA depletion and RNA missplicing contribute to neuronal vulnerability from loss of TDP-43. Nat Neurosci 2011; 14(4): 459-68.
9. Kim SH, Shanware NP, Bowler MJ et al.: Amyotrophic lateral sclerosis-associated proteins TDP-43 and FUS/TLS function in a common biochemical complex to co-regulate HDAC6 mRNA. J Biol Chem 2010; 285(44): 34097-105.
10. Matzke MA, Birchler JA: RNAi-mediated pathways in the nucleus. Nat Rev Genet 2005; 6(1): 24-35.
11. Kim DH, Rossi JJ: Strategies for silencing human disease using RNA interference. Nat Rev Genet 2007; 8(3): 173-84.
12. Pushparaj PN, Melendez AJ: Short interfering RNA (si RNA) as a novel therapeutic. Clinical Exp Pharm Physiol 2006; 33: 504-510.
13. Kuźma-Kozakiewicz M, Kwieciński H: New therapeutic targets for amyotrophic lateral sclerosis (ALS). Exp Opinion Therap Targ 2011; 15(2): 127-43.
14. Elbashir SM, Harboth J, Lendeckel W et al.: Duplexes of 21-nucleotide RNAs mediate RNA interference in cultured mammalian cells. Nature 2001; 411: 494-8.
15. Kumar M, Carmichael GG: Antisense RNA: function and fate of duplex RNA in cells of higher eukaryotes. Microbiol Mol Biol Rev 1998; 62(4): 1415-34.
16. Maxwell MM: RNA1 applications in therapy development for neurodegenerative disease. Curr Pharmaceutical Design 2009; 15: 3977-91.
17. Sledz CA, Holko M, de Veer MJ et al.: Activation of the interferon system by short-interfering RNAs. Nat Cell Biol 2003; 5(9): 834-9.
18. Kumar P, Wu H, McBride JL et al.: Transvascular delivery of small interfering RNA to the central nervous system. Nature 2007; 448(7149): 39-43.
19. Hadaczek P, Eberling JL, Pivirotto P et al.: Eight years of clinical improvement in MPTP-lesioned primates after gene therapy with AAV2-hAADC. Mol Ther 2010; 18: 1458-1461.
20. Grimm D, Streetz KL, Jopling CL et al.: Fatality in mice due to oversaturation of cellular microRNA/short hairpin RNA pathways. Nature 2006; 441(7092): 537-41.
21. Reid T, Warren R, Kirn D: Intravascular adenoviral agents in cancer patients: Lessons from clinical trials. Cancer Gene Ther 2002; 9: 979-86.
22. Raoul C, Abbas-Terki T, Bensadoun JC et al.: Lentiviral-mediated silencing of SOD1 through RNA interference retards disease onset and progression in a mouse model of ALS. Nat Med 2005; 11(4): 423-8.
23. Wang H, Ghosh A, Baigude H et al.: Therapeutic gene silencing delivered by a chemically modified small interfering RNA against mutant SOD1 slows amyotrophic lateral sclerosis progression. J Biol Chem 2008; 283(23): 15845-52.
24. Locatelli F, Corti S, Papadimitriou D et al.: Fas small interfering RNA reduces motoneuron death in amyotrophic lateral sclerosis mice. Ann Neurol 2007; 62(1): 81-92.
25. Ralph GS, Radcliffe PA, Day DM et al.: Silencing mutant SOD1 using RNAi protects against neurodegeneration and extends survival in an ALS model. Nat Med 2005; 11(4): 429-33.
26. Miller TM, Kaspar BK, Kops GJ et al.: Virus-delivered small RNA silencing sustains strength in amyotrophic lateral sclerosis. Ann Neurol 2005; 57(5): 773-776.
27. Miller T, Smith R, Kordasiewicz H et al.: Gene-targeted therapies for the central nervous system. Arch Neurol 2008; 65(4): 447-451.
28. Smith RA, Miller TM, Yamanaka K et al.: Antisense oligonucleotide therapy for neurodegenerative disease. J Clin Invest 2006; 116(8): 2290-6.
29. Crooke ST: Antisense Strategies. Current Molecular Medicine 2004; 4: 465-487.
30. Forte A, Cipollaro M, Cascino A et al.: Small interfering RNAs and antisense oligonucleotides for treatment of neurological diseases. Curr Drug Targets 2005; 6: 21-29.
31. Turner BJ, Cheah IK, Macfarlane KJ et al.: Antisense peptide nucleic acid-mediated knockdown of the p75 neurotrophin receptor delays motor neuron disease in mutant SOD1 transgenic mice. J Neurochem 2003; 87(3): 752-63.
32. Rembach A, Turner BJ, Bruce S et al.: Antisense peptide nucleic acid targeting GluR3 delays disease onset and progression in the SOD1 G93A mouse model of familial ALS. J Neurosci Res 2004; 77(4): 573-82.
33. Miller T, Smith R, Aggarwal S et al.: Cohort 1 of a phase 1, double-blind, placebo-controlled, dose-escalation study of the safety, tolerability, and pharmacokinetics of ISIS 333611 administered intrathecally to patients with familial ALS due to SOD1 gene mutations. AAN 2011; Honolulu, Hawaii; IN12-1.001.