© Borgis - Postępy Nauk Medycznych 12/2013, s. 856-864
Barbara Terelak-Borys, *Anna Walczak, Iwona Grabska-Liberek
Naczyniowe czynniki ryzyka w jaskrze normalnego ciśnienia i metody pomiaru ocznego przepływu krwi
Vascular risk factors in normal-tension glaucoma and techniques for evaluating ocular blood flow
Clinic of Ophthalmology, Medical Center of Postgraduate Education,
Prof. W. Orłowski Independent Public Clinical Hospital, Warszawa
Head of Clinic: Iwona Grabska-Liberek, MD, PhD, assoc. prof.
Streszczenie
Neuropatia jaskrowa jest najczęstszą neuropatią nerwu wzrokowego. Charakteryzuje ją postępująca utrata komórek zwojowych siatkówki i ich aksonów oraz uszkodzenie struktury tarczy nerwu wzrokowego, co skutkuje wystąpieniem ubytków w polu widzenia. Nieleczona prowadzi do nieodwracalnej utraty widzenia. Choroba ta jest – obok zwyrodnienia plamki związanego z wiekiem – najczęstszą przyczyną ślepoty w krajach cywilizowanych. Do niedawna diagnostyka i leczenie jaskry koncentrowały się zasadniczo na wykrywaniu i obniżaniu podwyższonego ciśnienia wewnątrzgałkowego, które stanowi główny czynnik ryzyka rozwoju jaskry. Jednakże u części pacjentów pomimo prawidłowych wartości ciśnienia wewnątrzgałkowego dochodzi do rozwoju i dalszej progresji neuropatii jaskrowej. Do tej grupy należą pacjenci z jaskrą normalnego ciśnienia. Według obecnego stanu wiedzy uznaje się, że jedną z zasadniczych przyczyn uszkodzenia nerwu wzrokowego w jaskrze jest mechanizm niedokrwienia: wtórnego do podwyższonego ciśnienia śródgałkowego lub pierwotnego, spowodowanego zaburzeniami ocznego przepływu krwi. Uważa się, że w jaskrze normalnego ciśnienia ciśnienie śródgałkowe nawet w granicach statystycznej normy jest za wysokie w stosunku do indywidualnej oporności struktury tarczy nerwu II. W tej postaci jaskry upośledzenie krążenia krwi w gałce ocznej bardzo często współistnieje z zaburzeniami hemodynamiki krążenia systemowego. Możliwe, że zaburzenia ukrwienia tarczy nerwu wzrokowego powodują wzmożoną podatność jego włókien na działanie ciśnienia śródgałkowego.
W niniejszym artykule zostaną omówione mechanizmy regulacji ocznego przepływu krwi oraz naczyniowe czynniki ryzyka, które mogą upośledzać krążenie systemowe i w gałce ocznej. W drugiej części pracy zostaną przedstawione metody wizualizacji i pomiaru ocznego przepływu krwi.
Summary
The most common optic neuropathy is the glaucomatous neuropathy. It involves progressive loss of retinal ganglion cells including their axons and disc damage resulting in visual field loss. The disease, if not treated, can lead to irreversible vision loss. Apart from the age-related macular degeneration glaucoma is the main cause of blindness in civilized countries. Until recently the diagnosis and treatment of glaucoma mainly focused on the detection and reduction of the intraocular pressure, which is found the main risk factor for glaucoma. However, in spite of the normal intraocular pressure some glaucoma patients develop the neuropathy and experience progression of the disease. These patients mostly suffer from the normal-tension glaucoma. According to the current state of knowledge it is believed that one of the leading causes of the optic disk damage in glaucoma is the ischemia of the secondary to elevated intraocular pressure or a disturbance of ocular blood flow. It is considered that the intraocular pressure within statistically normal limits is relatively too high for disk resistance in eyes with normal-tension glaucoma. In these patients the failure of blood circulation in eye usually co-exists with systemic hemodynamic alternations. It is also possible that the disturbances of blood supply to the optic nerve head may cause increased susceptibility of the retinal nerve fibres to the intraocular pressure.
The review discusses the mechanisms that (may) affect the regulation of the ocular blood flow and the vascular risk factors that may compromise the systemic and ocular blood flow. The second part of the article summarizes some new visualization techniques for evaluating ocular blood flow.
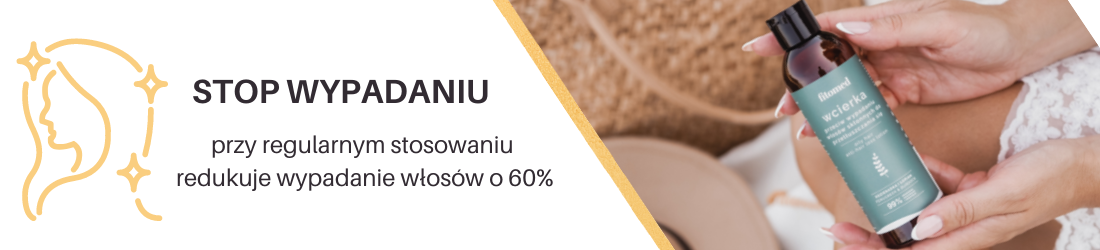
INTRODUCTION
Normal tension glaucoma (NTG) is a chronic optic neuropathy distinguished by a progressive loss of retinal ganglion cells along with their axons, and an alteration of the optic disc including the cribrum. These processes result in a loss of the field of vision. The term “normal tension glaucoma” was first used by A. von Graefe in 1857. Due to numerous similarities between the normal tension glaucoma and the primary open-angle glaucoma (POAG), i.e. open filtration angle, glaucomatous damage to the optic disc and visual field defects, normal tension glaucoma was acknowledged by most researchers, as well as by the European Glaucoma Society, as a variation of the primary open-angle glaucoma. The essential difference between these two forms of glaucoma is the value of intraocular pressure. Measurements of the diurnal curve of intraocular pressure in patients with a diagnosed NTG do not exceed 21 mmHg. It is advisable to exclude other causes of optic atrophy in the diagnosis confirmation, although traits of glaucomatous damage to the optic nerve, with a formation of a characteristic cup in its disc, are considered pathognomonic for this disease (1-4).
PREVALENCE
There are discrepancies regarding the prevalence of NTG. Many researches show that this disease occurs much more often than medical practice would indicate. Numerous studies report that almost 25-50% of primary open-angle glaucoma patients had normal intraocular pressure values during the first examination. In screening examinations of a group of glaucoma patients, 114 persons (58.8%) demonstrated an intraocular pressure value of less than 21 mmHg. Other authors have estimated that 20-30% of open-angle glaucoma patients suffer from NTG. In Japan, NTG patients account for more than 50% of all glaucoma patients – this disease occurs more frequently in this population than among people of Caucasian origin. It also occurs twice as often in women than in men (5).
ETIOPATHOGENESIS
It has been proven, both clinically and experimentally, that elevated intraocular pressure causes damage to the optic disc. In 1858, Müller presented a so-called mechanical theory of glaucoma, which was then modified over time. It is based on an assumption that elevated pressure in the eyeball (or relatively high as in the case of NTG) deforms the cribrum, exerts pressure on the optic nerve and causes disturbances in the axoplasmic flow, which leads to ganglion cells’ death. The second theory was proposed by von Jaeger, also in 1858 and is called the vascular theory. The premise of this theory is that the main cause of the development of glaucomatous neuropathy is generalized ischemia of the optic nerve (6, 7).
The fact that intraocular pressure in NTG persons remains within normal limits (statistically determined value of normal intraocular pressure) suggests that it can be harmful to the optic nerve. According to Yamamoto and Kitazawa, NTG can be defined as a glaucomatous neuropathy developing in eyes with normal intraocular pressure, for which eyes this pressure is relatively high and determines damage to the optic nerve (6). The Collaborative Normal Tension Glaucoma Study shows that a 30% reduction of intraocular pressure in patients with diagnosed NTG lowers the risk of progression from 35 to 12% (8). Cartwright, Anderson and Crichton indicate in their research that NTG patients with elevated intraocular pressure had larger visual field defects than patients of the same group but with lower values of the pressure in the eyeball (5).
It was postulated for many years that the main risk factor in the development and progression of glaucoma is high intraocular pressure. Numerous studies (Ocular Hypertension Study, Early Manifest Glaucoma Trial, Collaborative Initial Glaucoma Treatment Study and Advanced Glaucoma Intervention Study) document the principal role of intraocular pressure in the pathogenesis of glaucoma, however, whether it is the only damaging factor is still a matter of dispute (5, 9-12). In recent years, the role of vascular risk factors for glaucomatous neuropathy development has been highlighted, especially in the context of NTG. It seems that abnormalities in the ocular blood flow or in the vascular autoregulation can be a leading cause of glaucoma in many cases.
ANATOMY AND PHYSIOLOGY OF OCULAR BLOOD FLOW
The anatomy and physiology of the ocular circulation is quite complex. We can distinguish three basic ocular vascular systems: choroidal circulation, retinal circulation and optic disc circulation.
The choroidal circulation accounts for 85% of the total ocular flow (13). Posterior ciliary arteries, branches of the ophthalmic artery, are responsible for supplying the choroid. The choroid is extensively autonomically innervated. Its vessels are characterized by the presence of fenestration, which allows the hormones circulating in the blood, as well as mediators produced by the vascular endothelium, to penetrate the vascular wall. The choroid is distinguished by a high blood flow and low oxygen extraction. The choroidal flow plays an important role in the supply of nutrients for the external retinal layers and is responsible for maintaining constant temperature and volume of the eye.
Retinal vessels are supplied in blood by the central retinal artery which is a branch of the ophthalmic artery. The branches of the central retinal artery supply the inner retinal layers. Contrary to the choroid vessels, they are devoid from autonomic innervation and are not subject to influences of sympathetic and parasympathetic systems. Also, unlike the choroid vessels, tight junctions between retinal vascular endothelial cells create an inner “blood-retina” barrier. Likewise, unlike in the case of choroid circulation, the retinal circulation is characterized by a low level of blood flow and high oxygen extraction.
The superficial layers of the optic disc are supplied with blood by small branches ramifying directly from the central retinal artery. The prelaminar region of the optic disc is supplied by the choroidal arterioles and by short posterior ciliary arteries. Because the choroid adheres directly to the prelaminar segment of the optic disc, this region can be affected by vasomotor mediators from the choroid. The optic disc is a special region on the border of retina and central nervous system, where there is no proper “blood-retina” barrier or, in other words, a “blood-brain” barrier.
REGULATION MECHANISMS OF THE OCULAR BLOOD FLOW
Different systems are engaged in the regulation of ocular blood flow, namely the autonomous nervous system, vascular endothelial cells and hormones circulating in the serum.
Choroidal flow takes place mainly under the control of signals coming from the autonomous nervous system and from hormones circulating in the serum. Neurogenic control of the ocular blood flow involves affecting vessels of the substances released from the autonomous system nerve endings, including norepinehrine, acetylcholine, substance P, cholecystokinin, vasoactive intestinal peptide (VIP), nitric oxide (NO), neuropeptide Y and ATP. Hormonal control involves the influence of hormones in the serum such as angiotensin, epinephrine, vasopressin and natriuretic peptide. They indirectly influence the endothelial cells and affect the vascular smooth muscles and pericytes directly.
Due to the “blood-retinal” barrier and a lack of autonomic innervation, the retinal circulation is based on autoregulation mechanisms, associated mainly with the function of vascular endothelium. Moreover, the retinal circulation is also regulated by nerve and glial cells. Autoregulation means an inner ability of a tissue to maintain a relatively stable blood flow despite alternating perfusion pressure, or a capability of changing the flow according to metabolic demand (5). The process of autoregulation depends on myogenic response, metabolic mechanisms and functions of the vascular endothelium. The myogenic mechanism is activated when the vascular blood flow increases. The result is a change in the calcium concentration of the vascular smooth muscle cells and a contraction of the vessel, leading to lowering of the blood flow. This mechanism protects against hyperperfusion. Metabolic mechanism consists in a change of vascular wall tension, which is a result of the influence of metabolites and ions (such as oxygen, carbon dioxide, potassium ions, adenosine), which are contained in the extracellular fluid. The vascular endothelium is primarily an active regulator maintaining adequate vascular tension. This happens in the mechanism of stimuli (physical, chemical and biological) reception and responding, in the form of production and release of vasoactive factors. The substances released by the vascular endothelium are: NO, endothelin-1 (ET-1) and prostacyclines including the so-called PGI2. The NO affects the smooth muscles and pericytes, leading to vascular relaxation. ET-1 causes an opposite effect, mainly affecting its ETa receptors. In a lower concentration, also in ocular circulation, the ET-1 can stimulate the production of NO and PGI, by connecting with ETb receptor, consequently leading to vasodilatation.
An exceptional vascular system in the eyeball is the one which is connected with the optic disc. Although the flow on the level of these vessels is regulated by endothelial cells, the direct adherence of choroid to the prelaminar segment of the optic disc causes the neurotransmitters, systemic hormones and endothelial mediators from the peripapillary choroid (e.g. endothelin-1 or angiotensin 2) to affect the sinews of the optic nerve in a place strategic for their damage in glaucoma, what may cause certain clinical implications (13). In vivo studies have shown a drop in perfusion of the optic nerve as a result of angiotensin 2. The effect of these mediators on the vessels in the optic disc region remains to be explained (5, 14, 15). Their angiospastic influence is considered to lead to autoregulation disorders and a heightened susceptibility of the optic nerve’s sinews to the damaging activity of intraocular pressure.
DISTURBANCES OF OCULAR BLOOD FLOW AND THE DEVELOPMENT OF GLAUCOMATOUS NEUROPATHY
Studies, in which it has been observed that the field of vision in some of the glaucoma patients has improved after the application of calcium channel blockers, have indicated the role of vascular factors in glaucomatous neuropathy development. Numerous studies confirm that the ocular blood flow in glaucoma patients is impaired (5, 16, 17). It concerns the flows in the choroid, retina and optic disc. According to Gasser and Flammer, the reduction of ocular blood flow can be of primary character, especially in normal tension glaucoma. This is confirmed by the fact that the blood flow in NTG patients is decreased not only in the eye but also peripherally in the whole body. There are studies that confirm the existence of lowered ocular blood flow in a number of people, long before they develop glaucomatous neuropathy (3, 5).
The blood flow depends on the perfusion pressure, which is a difference between the arterial and venous pressure (in the eye, the latter levels out with the intraocular pressure), and on vascular resistance. The ocular blood flow can be disturbed in two situations: in case of intraocular or arterial pressure fluctuations exceeding the possibility of compensation through autoregulation, and in case of disorders of vascular wall tension. If the increase of intraocular pressure is too high or the systemic pressure drop is too severe, the efficiency of autoregulation (which depends on maximum possible dilation of the arterial vessel, so-called depletion of “autoregulation reserve”) may be exhausted and the blood flow may drop. The impairment of the autoregulation ability, which can lead to tissue ischemia, is connected with an increased rigidity (functional or anatomic) of the arterial wall. As a consequence, the vessel cannot relax properly – adequately to the oxygen requirement.
In recent years, the theory of glaucomatous neuropathy development resulting from lowered ocular blood flow has been changed. It has been observed that in persons with carotid artery closure the NTG occurs with similar frequency as in a control group of healthy people. In many patients with multiple sclerosis and reduced ocular blood flow resulting from a high level of ET-1 in the serum the glaucomatous neuropathy does not develop. In older NTG patients with a history of circulatory system diseases, especially atherosclerosis, with stably reduced blood flow in the optic nerve, a typical glaucomatous excavation of the optic nerve also does not develop, but an atrophic image of the optic disc does. The above mentioned examples led to creation of a new hypothesis in the vascular theory in glaucomatous neuropathy development. It seems that the development of glaucoma is connected with instability of ocular blood flow rather than with permanent ischemia of the optic nerve (2). Numerous studies seem to confirm this hypothesis: Baltimore Eye Survey, Barbados Eye Study, Barbados Incidence Study of Eye Diseases and Enga-Neumarkt Study (5, 18, 19).
VASCULAR RISK FACTORS FOR GLAUCOMA
Powyżej zamieściliśmy fragment artykułu, do którego możesz uzyskać pełny dostęp.
Mam kod dostępu
- Aby uzyskać płatny dostęp do pełnej treści powyższego artykułu albo wszystkich artykułów (w zależności od wybranej opcji), należy wprowadzić kod.
- Wprowadzając kod, akceptują Państwo treść Regulaminu oraz potwierdzają zapoznanie się z nim.
- Aby kupić kod proszę skorzystać z jednej z poniższych opcji.
Opcja #1
29 zł
Wybieram
- dostęp do tego artykułu
- dostęp na 7 dni
uzyskany kod musi być wprowadzony na stronie artykułu, do którego został wykupiony
Opcja #2
69 zł
Wybieram
- dostęp do tego i pozostałych ponad 7000 artykułów
- dostęp na 30 dni
- najpopularniejsza opcja
Opcja #3
129 zł
Wybieram
- dostęp do tego i pozostałych ponad 7000 artykułów
- dostęp na 90 dni
- oszczędzasz 78 zł
Piśmiennictwo
1. European Glaucoma Society: Terminology and guidelines for glaucoma. Berlin 2008.
2. Flammer J, Mozaffarieh M: What Is the Present Pathogenetic Concept of Glaucomatous Optic Neuropathy? Surv Ophthalmol 2007; 52: 162-173.
3. Pache M, Flammer J: A Sick Eye in a Sick Body? Systemic Findings in Patients with Primary Open-angle Glaucoma. Surv Ophthalmol 2006; 51: 179-212.
4. Sowka J: New thoughts on normal tension glaucoma. Optometry 2005; 76: 600-608.
5. Harris A, Moss A, Russia D et al.: Aktualne poglądy na jaskrę: naczyniowe czynniki ryzyka. Górnicki Wydawnictwo Medyczne, Wrocław 2010.
6. Yamamoto T, Kitazawa Y: Vascular Pathogenesis of Normal-tension Glaucoma: a Possible Pathogenetic Factor, Other than Intraocular pressure, of Glaucomatous Optic Neuropathy. Progress in Retinal and Eye Research 1998; 17: 127-143.
7. Kuprjanowicz L, Karczewicz D: Neuroprotekcja w jaskrze. Annales Academiae Medicae Stetinensis: Roczniki Pomorskiej Akademii Medycznej w Szczecinie 2007; 53: 22-29.
8. Collaborative Normal Tension Glaucoma Study Group: The effectiveness of intraocular pressure reduction in the treatment of normal tension glaucoma. Am J Ophthalmol 1998; 126: 498-505.
9. Kass MA, Heter DK, Higginbotham EJ et al.: The Ocular Hypertension Treatment Study: A randomized trial determines that topical ocular hypotensive medication delays or prevents the onset of primary open-angle glaucoma. Arch Ophthalmol 2002; 120: 701-713.
10. Early Manifest Glaucoma Trial Study Group, Heijl A, Leske MC et al.: Reduction of intraocular pressure and glaucoma progression: results from the Early Manifest Glaucoma Trial. Arch Ophthalmol 2002; 120: 1268-1279.
11. The Advanced Glaucoma Intervention Study: The relationship between control of intraocular pressure and visual field deterioration. The AGIS Investigators. Am J Ophthalmol 2000; 130: 429-440.
12. Musch D, Gillespie B, Lichter P et al.: Visual field progression in the Collaborative Initial Glaucoma Treatment Study: The impact of treatment and other baseline factors. Ophthalmology 2009; 116: 200-207.
13. Flammer J, Orgul S, Costa VP et al.: The impact of ocular blood flow in glaucoma. Progress in Retinal and Eye Research 2002; 21: 359-393.
14. Orgul S, Gugleta K, Flammer J: Physiology of Perfusion as It Relates to the Optic Nerve Head. Surv Ophthalmol 1999; 43: 17-26.
15. Grieshaber MC, Mozaffarieh M, Flammer J: What Is the Link Between Vascular Dysregulation and Glaucoma? Surv Ophthalmol 2007; 52: 144-154.
16. Ishida K, Yamamoto T, Kitazawa Y: Clinical factors associated with progression of normal-tension glaucoma. J Glaucoma 1998; 7: 372-377.
17. Koseki N, Araie M, Yamagami J et al.: Effects of oral brovincamine on Visual field damage In patients with normal-tension glaukoma with low-normal intraocular pressure. J Glaucoma 1999; 8: 117-123.
18. Leske MC, Conell AM, Wu SY et al.: Incidence of open-angle glaucoma: the Barbados Eye Studies. Arch Ophthalmol 2001; 119: 89-95.
19. Bonomi L, Marchini G, Marraffa M et al.: Vascular risk factors for primary open-angle glaucoma: the Egna-Neumarkt Study. Ophthalmology 2000; 107: 1287-1293.
20. Tokunaga T, Kashiwagi K, Tsumura T et al.: Association between nocturnal blond pressure reduction and progression of visual field defect in patients with primary open-angle glaucoma or normal-tension glaucoma. Jpn J Ophthalmol 2004; 48: 380-385.
21. Kashiwagi K, Hosaka O, Kashiwagi F et al.: Systemic circulatory parameters. Comparison between patients with normal tension glaucoma and normal subjects using ambulatory monitoring. Jpn J Ophthalmol 2001; 45: 388-396.
22. Suzuki Y, Iwase A, Araie M et al.: Risk factors for open-angle glaucoma in a Japanese population: the Tajimi Study. Ophthalmology 2006; 113: 1613-1617.
23. Beltrame JF, Sasayama S, Maseri A: Racial heterogeneity in coronary artery vasomotor reactivity: differences between Japanese and Caucasian patients. J Am Coll Cardiol 1999; 33: 1442-1452.
24. Flammer J, Pache M, Resink T: Vasospasm, its role in the pathogenesis of diseases with particular reference to the eye. Prog Retin Eye Res 2001; 20: 319-349.
25. Grieshaber MC, Terhorst T, Flammer J: The pathogenesis of optic disc splinter haemorrhages: a new hypothesis. Acta Ophthalmol Scand 2006; 84: 62-68.
26. Drance S, Anderson DR, Schulzer M et al.: Risk factors for progression of visual field abnormalities in normal-tension glaucoma. Am J Ophthalmol 2001; 131: 699-708.
27. Bojić L, Skare-Librenjak L: Circulating platelet aggregates in glaucoma. Int Ophthalmol 1998; 22: 151-154.
28. Matsumoto M, Matsuhashi H, Nakazawa M: Normal tension glaucoma and primary open angle glaucoma associated with increased platelet aggregation. Tohoku J Exp Med 2001; 193: 293-299.
29. Kremmer S, Kreuzfelder E, Klein R et al.: Antiphosphatidylserine antibodies are elevated in normal tension glaucoma. Clin Exp Immunol 2001; 125: 211-215.
30. Ellis JD, Evans JMM, Ruta DA et al.: Glaucoma incidence in an unselected cohort of diabetic patients: is diabetes mellitus a risk factor for glaucoma? Br J Ophthalmol 2000; 84: 1218-1224.
31. Quigley HA: Can Diabetes Be Good for Glaucoma? Why Can’t We Believe Our Own Eyes (or Data)? Arch Ophthalmol 2009; 127: 227-229.
32. Nishijima K, Ng YS, Zhong LC et al.: Vascular endothelial growth factor-A is a survival factor for retinal neurons and a critical neuroprotectant during the adaptive response to ischemic injury. Am J Pathol 2007; 171: 53-67.
33. Hosking SL, Evans DW, Embleton SJ et al.: Hypercapnia invokes an acute loss of contrast sensitivity in untreated glaucoma patients. Br J Ophthalmol 2001; 85: 1352-1356.
34. Palma BD, Gabriel A, Bignotto M et al.: Paradoxical sleep deprivation increases plasma endothelin levels. Braz J Med Biol Res 2002; 35: 75-79.
35. Haefliger IO, Dettmann E, Liu R et al.: Potential role of nitric oxide and endothelin in the pathogenesis of glaucoma. Surv Ophthalmol 1999; 43 (suppl. 1): 51-58.
36. Kirwan RP, Crean JK, Fenerty CH et al.: Effect of cyclical mechanical stretch and exogenous transforming growth factor-beta1on matrix metalloproteinase-2 activity in lamina cribrosa cells from the human optic nerve head. J Glaucoma 2004; 13: 327-334.
37. Gherghel D, Orgul S, Gugleta K et al.: Relationship between ocular perfusion pressure and retrobulbar blood flow in patients with glaucoma with progressive damage. Am J Ophthalmol 2000; 130: 597-605.
38. Cheng CY, Liu CJ, Chiou HJ et al.: Color Doppler imaging study of retrobulbar hemodynamics in chronic angle-closure glaucoma. Ophthalmology 2001; 108: 1445-1451.
39. Martinez A, Sanchez M: Predictive value of colour Doppler imaging in a prospective study of visual field progression in primary open-angle glaucoma. Acta Ophthalmologica Scandinavica 2005; 83: 716-722.
40. Ciancaglin M, Carpineto P, Costagliola C et al.: Perfusion of the optic nerve head and visual field damage in glaucomatous patients. Graefes Arch Clin Exp Ophthalmol 2001; 239: 271-277.
41. Chung HS, Harris A, Kagemann L et al.: Peripapillary retinal blood flow in normal tension glaucoma. Br J Ophthalmol 1999; 83: 466-469.
42. Lam A, Piltz-Seymour J, Dupont J et al.: Laser Doppler flowmetry in asymmetric glaucoma. Curr Eye Res 2005; 30: 221-227.
43. Sato EA, Ohtake Y, Shinoda K et al.: Decreased blood flow at neuroretinal rim of optic nerve head corresponds with visual field deficit in eyes with normal tension glaucoma. Graefes Arch for Clin and Exp Ophthalmol 2006; 244: 795-801.
44. Sugiyama T, Schwartz B, Takamoto T et al.: Evaluation of the circulation in the retina, peripapillary choroids and optic disk in normal-tension glaucoma. Ophthalmic Res 2000; 32: 79-86.
45. Duijm HF, Berg TJ, Greve EL: Central and peripheral arteriovenous passage times of the retina in glaucoma. Exp Eye Res 1999; 69: 145-153.
46. Arend O, Remky A, Redbrake C et al.: Retinal hemodynamics in patients with normal pressure glaucoma. Quantification with digital laser scanning fluorescein angiography. Ophthalmologe 1999; 96: 24-29.
47. Plange N, Remky A, Arend O: Absolute filling defects of the optic disc in fluorescein angiograms in glaucoma – a retrospective clinical study. Klin Monatsbl Augenheilkd 2001; 218: 214-221.
48. Schmetterer L, Dallinger S, Findl O et al.: A comparison between laser interferometric measurement of fundus pulsation and pneumotonometric measurement of pulsatile ocular blood flow. 1. Baseline considerations. Eye 2000; 14: 39-45.
49. Kerr J, Nelson P, O’Brien C: A comparison of ocular blood flow in untreated primary open-angle glaucoma and ocular hypertension. Am J Ophthalmol 1998; 126: 42-51.
50. Findl O, Rainer G, Dallinger S et al.: Assessment of optic disc blood flow in patients with open angle glaucoma. Am J Ophthalmol 2000; 130: 589-596.
51. Fuchsjäger-Mayrl G, Wally B, Georgopoulos M et al.: Ocular blood flow and systemic blood pressure in patients with primary openangle glaucoma and ocular hypertension. Invest Ophthalmol Vis Sci 2004; 45: 834-839.
52. Arend O, Remky A, Cantor L et al.: Altitudinal visual field asymmetry is couple with altered retinal circulation in patients with normal pressure glaucoma. Br J Ophthalmol 2000; 84: 1008-1012.
53. Schwenn O, Troost R, Vogel A et al.: Ocular pulse amplitude in patients with open angle glaucoma, normal tension glaucoma, and ocular hypertension. Br J Ophthalmol 2002; 86: 981-984.
54. Vulsteke C, Stalmans I, Fieuws S et al.: Correlation between ocular pulse amplitude measured by dynamic contour tonometer and visual field defects. Graefes Arch Clin Exp Ophthalmol 2008; 246: 559-565.
55. Schmidt KG, von Ruckmann A, Pillunat LE: Topical carbonic anhydrase inhibition increases ocular pulse amplitude in high tension primary open angle glaucoma. Br J Ophthalmol 1998; 82: 758-762.
56. Fontana L, Poinoosawmy D, Bunce CV et al.: Pulsatile ocular blood flow investigation in asymmetric normal tension glaucoma and normal subjects. Br J Ophthalmol 1998; 82: 731-736.
57. Findl O, Rainer G, Dallinger S et al.: Assessment of optic disk blood flow in patients with open-angle glaucoma. Am J Ophthalmol 2000; 130: 589-596.
58. Tamaki Y, Araie M, Hasegawa T et al.: Optic nerve head circulation after intraocular pressure reduction achieved by trabeculectomy. Ophthalmology 2001; 108: 627-632.
59. Wehbe HM, Ruggeri M, Jiao S et al.: Automatic retinal blood flow calculation using spectral domain optical coherence tomography. Opt Express 2007; 15: 15193-15206.
60. Harris A, Kagemann L, Ehrlich R et al.: Measuring and interpreting ocular blood flow and metabolism in glaucoma. Can J Ophthalmol 2008; 43: 328-336.