© Borgis - Postępy Nauk Medycznych 11/2015, s. 779-786
*Jacek Michałkiewicz1, 2
Udział mechanizmów odpornościowych w patogenezie pierwotnego nadciśnienia tętniczego
The role of immune system in pathogenesis of primary hypertension**
1Department of Microbiology and Immunology, The Children’s Memorial Health Institute, Warszawa
2Chair of Immunology, Collegium Medicum in Bydgoszcz, Nicolaus Copernicus University, Toruń
Streszczenie
Większość badań układu odpornościowego u ludzi z pierwotnym nadciśnieniem tętniczym wykonano u osób dorosłych, stąd ich znaczenie patogenetyczne jest wątpliwe z powodu licznych obciążeń, niezwiązanych bezpośrednio z chorobą pierwotną, takich jak stosowanie używek, leków, obecność zaburzeń metabolicznych i innych uwarunkowań, powodujących zwiększoną aktywację mechanizmów odpornościowych, manifestujących się przewagą limfocytów o fenotypie komórek efektorowych i pamięci immunologicznej (profil prozapalny), a mniejszą populacją limfocytów dziewiczych (profil niezapalny).
Dziecięca postać PN (nadciśnienie pierwotne) stanowi najbardziej przydatny model badawczy chorób sercowo-naczyniowych z powodu nieznacznego tylko obciążonia innymi czynnikami współistniejącymi. Zwiększenie ciśnienia tętniczego oraz zapalenie naczyń u dzieci z PN związane są z obecnością wykładników chronicznego, podostrego zapalenia systemowego, którego aktywność korelowała: a) ze zmianami aktywności metabolicznej tkanki tłuszczowej manifestującymi się wzrostem poziomu leptyny i spadkiem adiponektyny, b) ze zmianami w zakresie dystrybucji tkanki tłuszczowej, c) ze wzrostem stężenia surowiczych komponent stresu oksydacyjnego oraz niektórych cytokin prozapalnych.
Dzieci z PN charakteryzują się także: a) przyspieszeniem wieku biologicznego, wyrażonego jako różnica pomiędzy wiekiem kostnym a wiekiem chronologicznym, b) zaburzeniami w zakresie ekspresji genów systemu renina-angiotensyna oraz genów kontrolujących ekspresję receptorów dla adipokin i metaloproteinaz w leukocytach krwi obwodowej, c) zmianami w zakresie ekspresji szeregu receptorów komórkowych leukocytów takich jak AdipoR1, wzrostem populacji limfocytów o fenotypie komórek T-reg (regulatorowych) i ekspresją interleukiny 17.
Wyniki te wskazują, że PN u dzieci i dorosłych jest silnie związane z wieloma parametrami aktywacji układu odpornościowego, lecz pochodzenie czynnikow stymulujących ciągle pozostaje niewyjaśnione.
Summary
Most of human studies on immunity in hypertension have been performed in adults so their pathogenic significance still remains obscure because of the numerous confounders such as smoking, drugs use, metabolic disorders as well as generally high inflammatory background created by overall excess of the effector/memory lymphocyte populations over the naïve ones. PH children are regarded as the best clinical model of development of cardiovascular disease not influenced by other factors. Both blood pressure elevation and vascular inflammation in the PH children were associated with a systemic low-grade inflammation that correlated with: a) the changes in the metabolism of adipose tissue: increase in leptin and decline in adiponectin serum levels, b) altered distribution of fat tissue with relative increase of visceral fat over subcutaneous fat, c) serum elevation of oxidative stress components and certain pro-inflammatory cytokines. The PH children were also characterized by: a) accelerated biological maturation expressed as the difference between bone age and chronological age, b) changes in expression profile of genes of renin-angiotensin system and metalloproteinases as well as leukocyte surface markers such as AdipoR1 receptors, c) elevation of T-reg cell numbers and increase in transition of T-reg into Th17 cells. Altogether, these results strongly suggest that PH in both children and adults is strongly associated with immune cells activation but the origin of the stimulating agents still remain unknown.
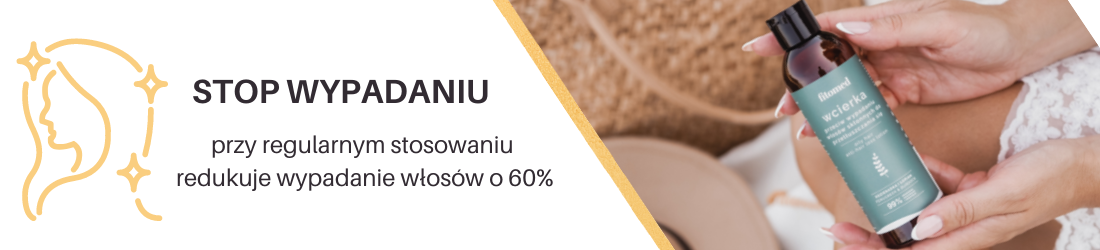
Introduction
The role of immune mechanisms in pathogenesis of hypertension was suggested by several early findings including: a) a functional thymus requirement for hypertension development, b) a presence of markers of systemic inflammation as well as agonistic antibodies against angiotensin II receptors and adrenergic receptors in the serum of hypertensive patients, c) a correction, amelioration or prevention of experimental hypertension by suppression of T cell driven inflammation in the target organs.
At present, primary hypertension (PH) both in humans and animal models is considered as a condition of low-grade, chronic, systemic inflammation often associated with metabolic syndrome components (dyslipidemia, insulin resistance, obesity, etc.), and acute phase response manifested by elevation of cytokines (IL-6, TNF-alfa) and C-reactive protein serum levels (1-4). Blood pressure elevation in the course of hypertension depends on innate and adaptive immune immune responses that result in inflammation in the kidney, arteries and central nervous system.
Innate immunity in hypertension
The innate immune system is responsible for fast and non-specific immediate inflammatory response recruited to eliminate infection or in response to tissue injury. The system consists of cells (granulocytes, monocytes, macrophages, dendritic cells, mast cells, NK lymphocytes) and many soluble factors (interferons, acute phase proteins, cytokines, chemokines, defensins, and complement fragments).
The cells of innate immune system express pattern recognition receptors (PRRs) which recognize pathogen associated molecular patterns (PAMPs) that are expressed and shared by large groups of pathogens or damage associated molecular patterns (DAMPs) that represent structures of damaged cells.
Dendritic cells (DCs) infiltrate the kidney and arterial walls in many hypertension models. Their numbers increase in perivascular tissue and adventitia in large vessels (aorta) and in medium sized vessels (mesenteric arteries) together with other immune cells including CD4+ and CD8+ T cells and macrophages. In the kidney, immune cells are located preferentially around renal arteries. The reason why DCs and other immune cells accumulate in peri-vascular space are unknown but possibly sympathetic nerve endings present in these areas play some role in this phenomenon (5). DCs are the most efficient antigen-presenting cells and promote a differentiation of T cells towards many different functional phenotypes (6). The number of activated DCs in perivascular space increased in angiotensin II and DOCA-salt hypertension. They expressed high levels of several co-stimulatory molecules engaged in antigen presentation to T cells (7).
Co-stimulators are the molecules that are engaged in providing the second signals necessary for optimal T cells activation following their stimulation by the first signals (the ones generated by recognition of antigens presented to T cells by DCs, macrophages and B cells which act as antigen-presenting cells – APCs). One of the best characterized co-stimulatory pathway is the CD28/B7 in which CD28 represents a surface protein constitutively expressed by over 90% of mature CD4+ T cells and about 50-70% of CD8+ T cells. The CD28 molecule interacts with its B7 ligands (CD80 and CD86) expressed by activated and/or resting APCs, respectively). Pharmacological inhibition of CD28/CD80 pathway, lack of this pathway in CD80/CD86 knock-out mice or engrafting wild type bone marrow in CD80/CD86 deficient mice restored the hypertensive response to angiotensin II (7).
It has recently been found that DCs played a causal role in hypertension development by increased formation of ROS and oxidative modifications of proteins by highly reactive gamma-ketoaldehydes (isoketals). DCs from angiotensin II infused mice produced increased amounts of O2-, accumulated isoketal protein adducts, and released cytokines such as IL-6, IL-1 beta and IL-23 and had elevated expression of costimulators such as CD80, CD86. They promoted T cell proliferation, especially CD8+, and polarized T cells to an inflammatory phenotype involving production of IL-17, IFN-gamma and TNF-alfa. DCs from hypertensive mice primed a hypertensive response in recipient mice to low-dose of angiotensin II.
Scavenging isoketals prevented these parameters of DCs activation and ameliorated hypertension. Exposure of DCs to the prooxidant agent tert-butyl hydroperoxide (t-BHP) promoted their ability to suport CD8+ T cell proliferation and hypertension, thus mimicking the effect of angiotensin II in vivo. Likewise, DCs pulsed with isoketal-modified proteins from renal homogenates potently stimulated T cell proliferation, while DCs pulsed with other oxidized lipid products did not. The authors also noted that plasma F-2 izoprostanes which are formed in concert with isoketals are elevated in humans with treated hypertension and very markedly elevated in patients with resistant hypertension. Isoketal-modified proteins were also elevated in circulating monocytes and DCs from human with hypertension and that these increased with severity of hypertension. The authors conclude that hypertensive stimuli activates DCs by promoting the formation of isoketals and suggest that reducing isoketals has potential as a treatment strategy for this disease (8).
These observations directly suport the earlier assumption that vascular inflammation that results in hypertension is initiated by neo-antigens formation in the target tissues such as vasculature and kidney. These antigens are subsequently recognized by macrophages and dendritic cells via PRRs. Antigen presentation to responder T cells (in draining lymph nodes) results in their further activation, proliferation and differentiation into numerous types of different effectors, responsible for vascular inflammation (9).
This scheme implies the role of both innate (macrophages, dendritic cells) and adaptive immunity (T cells) in hypertension development. These data also imply the development of memory adaptive immune responses to vascular neoantigens (autantigens) that develop in the onset of the disease that support the idea of autoimmune reactivities against certain vascular antigens, e.g. HSP-70 proteins (10, 11).
Macrophages and monocytes
Macrophages like DCs infiltrate the kidney and periadventitial areas of the peripheral vessels (aorta and medium sized arteries). Hypertension induced infiltration of monocytes/macrophages into the vessel wall, brain, heart and kidney represents the main component of vascular inflammation and inflammation-induced organ damage in many experimental models including polygenic, monogenic, renovascular and mineralocorticoid hypertension. Macrophage infiltration in the kidney appears to be most prominent in forms of hypertension associated with an activated renin-angiotensin-aldosteron system.
On the contrary, a reduction in macrophage infiltration is associated with improvement of hypertension in several animal models including spontaneously hypertensive rats (SHRs) (12), Dahl salt-sensitive rats (13), hypertension induced by angiotensin II (14) and aldosteron (15), salt-dependent hypertension (16) and autoimmune hypertensive renal disease (17).
Elimination of circulating monocytes in mouse model by action of diphtheria toxin (18), deficiency in macrophage population in osteopetrotic mice with mutation in colony – stimulating factor gene (19) and treatment of mice with the CCR2 or MCP-1 antagonists that blocks chemokine receptors (CCR-2) or chemokine itself (MCP-1), protected mice from angiotensin II or DOCA induced hypertension (20, 21).
Macrophages are also main targets for adrenergic and cholinergic regulations of the immune reactions in course of hypertension. The spleen macrophages of spontaneously hypertensive rats (SHRs) primed with nicotine (cholinergic, anti-inflammatory signal) or angiotensin II (pro-inflammatory signal) remain highly reactive in response to pro-inflammatory activities induced by second stimulation via certain TLRs (TLR-7,8) as measured by IL-6, and TNF-alfa production. These effects are observed only in the hypertensive rats but not in the control, normotensive rats, and appeared before the onset of hypertension indicating on impaired response to cholinergic signals in the pre-hypertension stage (22).
There is still unclear if macrophage infiltration into vessel walls or target organs represents “primary” or “secondary” alterations in the immune system. So far unidentified “primary” changes of the immune system may result in subsequent inflammatory response in the vessel wall and/or the kidney vasculature leading then to blood pressure elevation. There are some data suggesting that certain immune defects may preceed hypertension development in SHRs (23) but this remains difficult to confirm in human.
“Secondary” leukocyte alterations induced either by hypertension per se (via mechanical stress) and/or by factors that cause hypertension (e.g. angiotensin II) may also lead to the infiltration and/or activation of leukocytes, particularly macrophages, in target tissues where these cells contribute to the development of organ injury by realeasing pro-inflammatory agents including soluble mediators (cytokines, chemokines, oxygen radicals, etc.) and acting as cytotoxic cells for many vascular targets or other toxic mediators (24, 25).
From the practical reasons, the pathological diagnosis of hypertensive kidney disease is difficult to study in patients with hypertension. Macrophage analysis in atherectomy speciments is often confounded by the presence of atherosclerosis. Kidney biopsies may not be representative if the infiltration is as focal as it is in experimental hypertension (26). Diagnosis is often doubted by pathologists and a marked macrophage infiltration may even suggest the presence of other primary immunological kidney disease. A true “control” tissue without hypertension or atherosclerosis is hard to obtain. Therefore studies in human patients have been performed with the use of peripheral blood monocytes. There is a lot of data concerning this issue. In general, they indicated that hypertension was associated with the presence of activated monocytes in periphery, which showed: a) decreased sensitivity to blocking action of glucorticosteroids in vitro (27), b) increased adherence to endothelium in vitro and elevated production of IL-1 and TNF-alfa (28), TGF-beta (29), MCP-1 as well as TF (30) upon stimulation, c) elevated expression of some surface receptors related to endothelial adhesion and transmigration, CD11b, CD11c, ICAM-1, CCR2 and CCR-5 (31), d) increased expression of TLR-4 but not TLR-2 (32), e) increase in ROS production (33), f) increased expression of transient receptor potential canonical type 3 channels (TRPC3) that was associated with increased monocyte migration (34), g) up-regulation of bradykinine receptors 1 and 2 (35).
In addition, monocytes have some phenotype and functional characteristics which are strictly related to their role in blood pressure regulation. They inlude: a) expression of AT1 receptor for angiotensin II (36); it enables monocyte activation by this peptide that results in high production of several pro-inflammatory cytokines and has an influence on several monocyte functions related to their endothelial transmigration and differentiation to tissue macrophages, b) expression of renin angiotensinogen, and aldosteron (37), c) expression of adrenergic and cholinergic receptors which enable monocyte interaction with neurotransmitters (acetylocholina, noradrenaline, dopamine) that are engaged in blood pressure regulation (38) as well as abilities for their production (39) d) expression of leptin, and adiponectin receptors that enable monocytes to interact with these adipokines (40, 41).
The latter point is especially interesting. Fat mass is linked mechanistically to the cardiovascular system through adipokines action, including leptin. Leptin increases blood pressure via hypothalmic-sympathetic pathways and also stimulates many pro-inflammatory activities such as monocyte migration through extracellular matrix, up-regulation of monocyte scavenger receptor expression, increased uptake of oxidized low-density lipoprotein (LDL), cytokines secretion, adhesion to endothelium and other immune functions that contribute to vascular inflammation.
Powyżej zamieściliśmy fragment artykułu, do którego możesz uzyskać pełny dostęp.
Mam kod dostępu
- Aby uzyskać płatny dostęp do pełnej treści powyższego artykułu albo wszystkich artykułów (w zależności od wybranej opcji), należy wprowadzić kod.
- Wprowadzając kod, akceptują Państwo treść Regulaminu oraz potwierdzają zapoznanie się z nim.
- Aby kupić kod proszę skorzystać z jednej z poniższych opcji.
Opcja #1
29 zł
Wybieram
- dostęp do tego artykułu
- dostęp na 7 dni
uzyskany kod musi być wprowadzony na stronie artykułu, do którego został wykupiony
Opcja #2
69 zł
Wybieram
- dostęp do tego i pozostałych ponad 7000 artykułów
- dostęp na 30 dni
- najpopularniejsza opcja
Opcja #3
129 zł
Wybieram
- dostęp do tego i pozostałych ponad 7000 artykułów
- dostęp na 90 dni
- oszczędzasz 78 zł
Piśmiennictwo
1. Chae CU, Lee RT, Ritta N, Reidker PM: Blood pressure and inflammation in apparantly healthy man. Hypertension 2001; 38: 399-403.
2. Engstroem G, Janzon I, Berglund G et al.: Blood pressure increase and incidence of hypertension in relation to inflammation sensitive plasma proteins. Arterioscler Thromb Vasc Biol 2002; 22: 2054-2058.
3. Litwin M, Michalkiewicz J, Niemirska A et al.: Inflammatory activation in children with primary hypertension. Pediatr Nephrol 2010; 25: 2489-2499.
4. Litwin M, Sladowska J, Antoniewicz J et al.: Metabolic abnormalities, insulin resistance, and metabolic syndrome in children with primary hypertension. Am J Hypertens 2007; 20: 875-882.
5. Imig JD, Ryan NJ: Immune and inflammatory role in renal disease. Compr Physiol 2013; 2: 957-976.
6. Steinman RM, Hemmi H: Dedritic cells: translating innate to adaptive immunity. Curr Top Microbiol Immunol 2006; 311: 17-58.
7. Vinh A, Chen W, Blinder Y et al.: Inhibition and genetic ablation of the B7/CD28 T-cell costimulation axis prevents experimental hypertension. Circulation 2010; 122(24): 2529-2537.
8. Kirabo A, Fontana V, de Faria APC et al.: DC isoketal modified proteins activate T cells and promote hypertension. J Clin Invest 2014; 24(10): 4642-4656.
9. Trott WD, Harrison DG: The immune system in hypertension. Adv Physiol Edu 2014; 38: 20-24.
10. Pober JS: Is hypertension an autoimmune disease? J Clin Invest 2014; 24(10): 4234-4235.
11. Rodriguez-Iturbe B, Pons H, Quiroz Y et al.: Autoimmunity in the pathogenesis of hypertension. Immunity to HSP-70 over-expression in arteries and kidneys is involved in pathogenesis of salt-induced experimental hypertension and is considered as autoimmune phenomenon. Nature Reviews Nephrology 2014; 10: 56-62.
12. Rodriguez-Iturbe B, Quiroz Y, Nava M et al.: Reduction of renal immune cell infiltration results in blood pressure control in genetically hypertensive rats. Am J Physiol 2002; 282: F191-F201.
13. DeMiguel C, Das S, Lund H, Matson DL: T lymphocyte mediate hypertension and kidney damage in Dahl salt sensitive rats. Am J Physiol Regul Inegr Comp Physiol 2010; 298: R1136-R1142.
14. Muller DN, Shagarsuren E, Park JK et al.: Immunosuppressive treatment protects against angitensin II induced renal damage. Am J Pathol 2002; 161: 1679-1692.
15. Boesen H, Williams DL, Pollock JS, Pollock DM: Immunosuppression with mycophenolate mofetil attenuates the development of hypertension and albuminuria in deoxycorticosterone acetale-salt hypertensive rats. Clin Exp Pharmacol Physiol 2010; 37: 1106-1022.
16. Franco M, Martinez F, Quiroz Y et al.: Renal angiotensin II contentration and interstistial infiltration of immune cells are correlated with blood pressure levels and salt-sensitive hypertension. Am J Physiol Reg Integr Comp Physiol 2007; 293: R251-R256.
17. Venegas Pont M, Sartori-Valinotti JC, Maric C et al.: Rosiglitazone decreases blood pressure and renal injury in a female Mouse model of systemie lapus erythematosus. Am J Physiol Regul Inegr Comp Physiol 2009; 296: R11282-R11289.
18. Wenzel P, Knorr M, Kossmann S et al.: Lyzozyme M positive monocytes mediate angiotensin II-arterial hypertension and vascular dysfunction. Circulation 2011; 124: 1370-1381.
19. De Cinceis C, Amiri F, Brassard P et al.: Reduced vascular remodelling, endothelial dysfunction, and oxidative stress in resistance arteries of angiotensin II-infuseded macrophage colony stimulating factor – deficient mice: evidence for a role in inflammation in angiotensin II-induced vascular injury. Arterioscle Thromb Vasc Biol 2005; 25: 2106-2118.
20. Chan CI, Moore JP, Budzyn R et al.: Reversal of vascular macrophage accumulation and hypertension by a CCR2 antagonist in dexoxycorticosteron/salt treated mice. Hypertension 2012; 60: 1207-1212.
21. Elmaralby AA, Ouigley JE, Olearczyk JJ et al.: Chemokine receptor 2b inhibition provides renal protection in angiotensin II-salt hypertension. Hypertension 2007; 50: 1069-1076.
22. Harwani SC, Chapleau MW, Legge KL et al.: Neurohormonal modulation of the innate immune system is proinflammatory in the prehypetensive spontaneously hypertensive rat, a genetic model of Essentials hypertension. Hypertension 2011; 57: 469-476.
23. Norman RH, Dzielak DI, Bost KL et al.: Immune system dysfunction contributes to the aetiology of spontaneous hypertension. J Hypertension 1985; 3: 261-268.
24. Fu ML: Do immune system changes have a role in hypertension? J Hypertension 1995; 13: 1259-1265.
25. Hilgers KF: Monocyte/macrophages in hypertension. J Hypertension 2002; 20: 593-596.
26. Hilgers KF, Hartner A, Porst M et al.: Monocyte chemoattractant protein-1 and macrophage infiltration in hypertensive kidney injury. Kidney International 2000; 58: 2408-2419.
27. Wirtz PH, von Kanel R, Frey K et al.: Glucocorticoid sensitivity of circulating monocytes in Essentials hypertension. Am J Hypertension 2004; 6: 489-494.
28. Dorfel Y, Latsch C, Stuhlmuller B et al.: Preactivated peripheral blood monocytes in patients with Essentials hypertension. Hypertension 1999; 43: 113-117.
29. Porecca E, Di Fabbo C, Minclone G et al.: Increased trans forming growth factor beta production and gene expression by peripheral blood monocytes of hypertensive patients. Hypertension 1997; 30: 134-139.
30. Sardo MA, Campo SC, Mandraffino G et al.: Tissue factor and monocyte chemoatttractant protein-1 expression in hypersensive individuals with normal or increased carotid infima-media wall thickness. Clinical Chemistry 2008; 54: 5814-5823.
31. Syrbe M, Moebes A, Scholtze J et al.: Effect of angiotensin II type I receptor antagonist telmisartan on monocyte adhesion and activation in patients with essential hypertension. Hypertens Res 2007; 30: 521-528.
32. Marketou ME, Kontaraki JE, Evangelos A et al.: TLR-2 and TLR-4 gene expression in peripheral monocytes in non-diabetic hypertensive patients: the effect of intensive blood pressure lowering. J Clin Hypertension (Geenwich) 2012; 14: 330-335.
33. Lim HS, Patel JV, Lip G et al.: Reactive oxygen species production by circulating monocytes: insights from patophysiology to clinical hypertension. J Hum Hypertens 2006; 20: 307-309.
34. Zhao Z, Ni Y, Chen J et al.: Increased migration of monocytes in essential hypertension is associated with increased transient receptor potential channel canonical type 3 channels. PLoS 2012; 7(3): 1-13.
35. Marketou ME, Kontraki J, Zacharis E et al.: Differential gene expression of bradykinin receptors 1 and 2 in peripheral monocytes from patients with essential hypertension. J Human Hypertension 2014; 28(7): 450-455.
36. Rasini E, Cosentino M, Marino F et al.: Angiotensin II type 1 receptor expression in human leukocyte subsets. A flow cytometry and RT-PCR study. Regul Pept 2006; 134: 69-74.
37. Okamura A, Rakugi H, Ohishi M et al.: up-regulation of renin-angiotensin system during differentiation of monocytes to macrophages. J Hypertens 1999; 17: 537-545.
38. Marino F, Cosentino M: Adrenergic modulation of of immune cells: an update. Amino Acids 2013: 45-71.
39. Marino F, Cosentino M, Bombelli R et al.: Endogenous catecholamine synthesis, metabolizm, storage and uptake in human peripheral blood mononuclear cells. Exp Hematol 1999; 27: 489-495.
40. Cannon JG, Sharma G, Sloan G et al.: Leptin regulates CD16 expression in human monocytes in a sex specific manner. Physiol Rep 2014; 2(10): 1-14.
41. Litwin M, Sladowska J, Antoniewicz et al.: Metabolic abnormalities, insulin resistance and metabolic syndrome in children with primary hypertension. The American Journal of Hypertension 2007; 8: 875-882.
42. Litwin M, Feber J, Niemirska A, Michałkiewicz J: Primary hypertension is a disease of premature vascular aging associated with neuro-immuno-metabolic abnormalities. Pediatr Nephrol 2015 Feb 28 [Epub ahead of print].
43. Litwin M, Michałkiewicz J, Gackowska L: Primary hypertension in children and adolescents is an immuno-metabolic disease with hemodynamic conequences. Curr Hypertens Rep 2013; 15: 331-339.
44. Pang TTL, Narendran P: The distribtution of adiponectin receptors on human peripheral mononuclear cells. Ann New York Academy Sciences 2008; 1150: 143-145.
45. Gackowska L, Litwin M, Trojanek J et al.: Expression of adiponectin receptors on peripheral blood leukocytes of hypersetensive children is associated with the severity of hypertension. BioMed Research International 2015, in press.
46. Madjid M, Awan J, Willerson T, Casscells SW: Leukocyte count and coronary heart disease: implications for risk assessement. J Am Coll Cardiol 2004; 44(10): 1945-1956.
47. Fardon NJM, Wilkinson R, Thomas TH: Rapid fusion of granules with neutrophil cell membranes in hypertensive patients may increase vascular damage. Am J Hypertension 2001; 14(9): 927-933.
48. Harrison DG: The immune system in hypertension. Transaction of the American Clinical and Climatological Association 2014; 125: 130-140.
49. Mahdur MS, Harrison DG: Synapses, Signals, and cytokines: Interaction of the autonomic nervous system and immunity in hypertension. Circ Res 2012; 111: 1113-1116.
50. Abdoud FM, Harwani SC, Chapleau MW: Autonomic neural regulation of the immune system: implications for hypertension and cardiovascular disease. Hypertension 2012; 59: 755-762.
51. Niemirska A, Litwin M, Feber J, Jurkiewicz E: Blood pressure rhytmicity and visceral fat in children with hypertension. Hypertension 2013; 62: 782-788.
52. Litwin M, Simonetti GD, Niemirska A et al.: Altered cardiovascular rhytmicity in children with white coat and ambulatory hypertension. Pediatr Res 2010; 67: 419-423.
53. Schiffrin EL: The immune system: Role in hypertension. Canadian Journal of Cardiology 2013; 29: 543-548.
54. Wang J, Chen J, Chen C et al.: Elevated Th17 and IL-23 in hypertensive patients with acutely increased blood pressure. A J Immunology 2012; 8(2): 27-32.
55. Barhoumi T, Kasal DA, Li MW et al.: T regulatory lymphocytes prevent angiotensin II-induced hypertension and vascular injury. Hypertension 2011; 57: 469-476.
56. Vakan H, Kleinewietfeld M, Quadri F et al.: Regulatory T cells ameliorate angiotensin cardiac damage. Circulation 2009; 119(22): 2904-2912.
57. Kanellakis P, Dinh TN, Agrotis A, Bobil A: CD4+CD25+FoxP3+ regulatory T cells suppress cardiac fibrosis in the hypertensive heart. J Hypertens 2011; 29(9): 1820-1828.
58. Kassan M, Wecker A, Kadowitz P et al.: CD4+CD25+FoxP3 regulatory T cells and vascular dysfunction in hypertension. J Hypertens 2013; 31(10): 1939-1943.
59. Jevallee H, Tang TT, Cheng XR: Regulatory T cells and cardiovascular diseases. N A J Med Sci 2011; 4(4): 178-182.
60. Schiffrin E: Immune mechanisms in hypertension: How do regulatory T lymphocytes fit in? J Hypertens 2013; 31: 1944-1945.
61. Zhang W, Wang W, You H et al.: Interleukin 6 underlines angiotensin II induced hypertension and chronic renal damage. Hypertension 2012; 59: 136-144.
62. Samassekou O, Gajdi M, Drouin R, Yan J: Sizing the ends: normal length of human telomers. Ann Anat 2010; 192(5): 284-291.
63. Maue AC, Yager EJ, Swain SL et al.: T-cell immunosenescence: lessons learned from mouse models of aging. Trends in Immunolog 2009; 30(7): 301-305.
64. Maue AC, Haynes L: CD4 T cells and immunosenescence – a mini review. Gastroenterology 2009; 55(5): 491-495.
65. Larbi A, Fulop T, Pawelec G: Immune receptor signalling, aging and autoimmunity. Advances in Experimental Medicine and Biology 2008; 640: 312-324.
66. Youn JC, Yu HT, Lim BJ et al.: Immunosenescent CD8 T cells and CXC chemokine receptor type 3 receptor chemokines are increased in hyman hypertension. Hypertension 2013; 62: 126-133.
67. Tae Hee, Shin EC: T cell immunosenescence, hypertension, and arterial stiffness. Epidemiology and Health 2014; 36: e2014005.
68. Mahdur MS, Harrison DG: Senescent T cells and hypertension. New ideas about old cells. Hypertension 2013; 62: 13-15.
69. Zhou X, Chen K, Lei H, Sun Z: Klotho gene deficiency causes salt-sensitive hypertension via monocyte chemotactic protein-1 /CC chemokine receptor 2-mediated inflammation. JASN 2015; 26(1): 121-132.